What are the implications of perfusion on brain health? Simon Mills explores cerebral development, circulation and disease and invigorates our approach to mitigating neuroinflammation and its consequences.
Putting the pieces back together
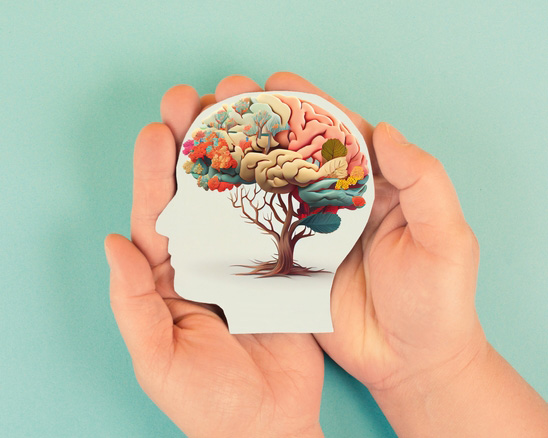
In modern times, Western herbal medicine has had a problem with the brain. In our atomised culture the body tissues and organs have become separated. At school we learnt that the kidneys do their things, the liver does others, the heart is a solitary pump, the lungs are bellows, and the brain is sealed off from the rest of the body —managing its substance the preserve of neurologists, its activity that of psychologists, and prescriptions largely restricted to neurotransmitter modulators.
Physiology has moved on of course, and in theory all the old barriers are down. For example, the liver and kidneys are wholly enmeshed, the heart is better understood as a resonator of the wider vascular pulsation, and the lungs, once a branch of the gut, become an intrinsic part of the circulatory and immune systems. We now understand the brain is dynamically interactive with the rest of the body, as evidenced by concepts like the gut-brain axis and psychoneuroendocrinology.
Medical practice is still however relatively fragmentary, and the old Cartesian reductionist language unfortunately still infects even our herbal discourse (what do we really mean by ‘nervines’?). Other herbal traditions never faced this challenge. For example, in Chinese traditional medicine organs are physical manifestations of vital functions (although in Western translations these functions are also misleadingly referred to as ‘organs’). So, for example, the brain is considered an extension of bone marrow and comes within the orbit of the Kidney function (shen), and thus is one manifestation of the most powerful energetic phenomena in the living being, along with bones, birth, development and reproduction. On the other hand, the brain’s activities, such as perception and cognition, are manifestations of the Heart function (xin) and are associated, therefore, variously with pleasure, our sense of self, and the dynamic aspects of circulation (1). These insights arise out of extensive deep reflections on human experiences over many centuries rather than dissection science. Arguably, they have renewed relevance as we discover the limitations of medical fragmentation in understanding complex health conditions.
Fortunately, new insights into brain and mental functions may allow us at last to rethink our therapeutic approaches in Western terms, and unlock the brain from its sealed cavity. In particular, we can see that many problems in this area are essentially vascular, and by extension potentially of inflammatory origin. We can look first at the close links central nervous tissues have with the blood circulation.
The practitioner can be encouraged to look with renewed interest on any remedy currently used for its circulatory effects with renewed interest as potential ‘new nervines’!
The brain and blood vessels start out together
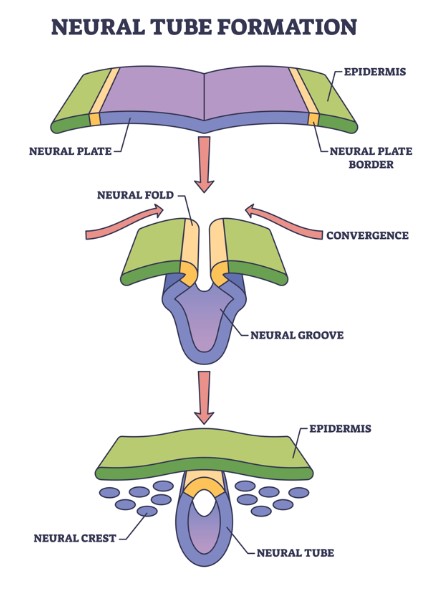
During embryogenesis and early life, the nervous and vascular systems intricately coordinate their development from common origins in the embryonic neural tube, itself derived from a fold in the ectoderm. Neurons and glia are derived from neural tube neuroectodermal stem cells, whereas specialised vascular components, including adapted smooth muscle cells called pericytes, are derived from the neural crest, a segregated portion of the neural tube.
There are no resident vascular precursors among the cells that will form the central nervous system, so vessel sprouts invade the developing brain and spinal cord at the outset, in a form of angiogenesis — the formation of new blood vessels (2). Once there, they follow cues provided by the developing nervous system to migrate alongside radial glia, neural progenitor cells that form long cell extensions along the neural tube. At medial surfaces (called the ventricular zone) radial glial cells undergo mitosis into daughter cells that migrate to the lateral surface of the tube and differentiate into neurons, microglia, the macroglia astrocytes, oligodendrocytes and ependymal cells, while others remain as stem cells. Invading vessel sprouts initially move to the border of the ventricular zone, but leave the radial glia there and migrate laterally, to form interconnections with the new cells (3).
In early neurovascular crosstalk, astrocytes link vascular endothelial cells and their attached pericytes to form intimate associations with the neurons, (4) particularly in embryological zones called ‘vascular niches’. It even appears that angiogenesis and neurogenesis are regulated by shared growth factors, released both by endothelial cells and neurons. These include members of the vascular endothelial growth factor (VEGF —see below), semaphorin, Wnt and integrin families, and neuropilin (5,6).
Oligodendrocytes, critical to myelination and remyelination of neurons, also migrate through the developing CNS along with blood vessels (7).
Neurovascular communication is the basis for functional magnetic resonance imaging (fMRI) scans, a technique that reveals changes in local blood flow and oxygenation that correlate with immediate and localised neural activity in the brain.
The neurovascular unit and blood-brain barrier
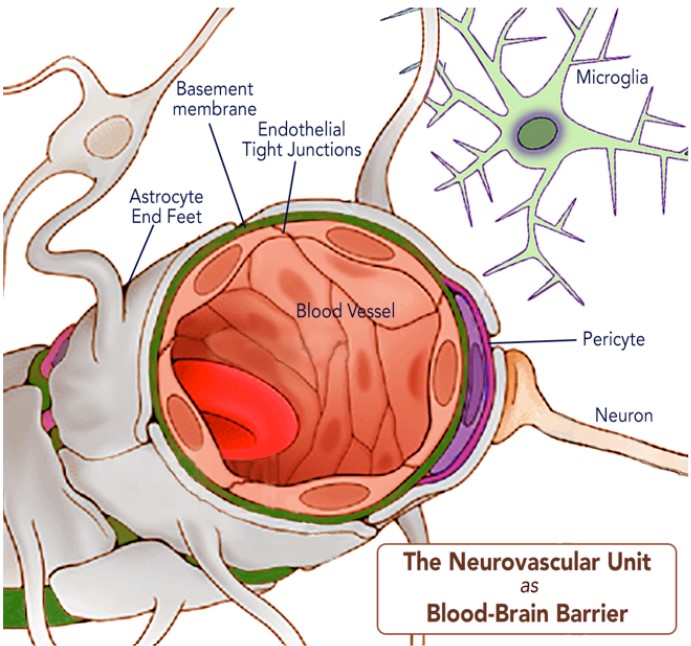
The co-development of cells in the nervous and circulatory systems reaches its most important and sophisticated manifestation where the two tissues interact in adult life. There is an immediate imperative for this to work well: over 20% of cardiac output is distributed to the brain to meet oxygen and glucose requirements, despite it only accounting for 2% of the body’s mass. To deliver adequate blood flow to neuronal and glial cells, the mammalian brain has evolved the neurovascular unit (NVU), a complex unit of cells that connects the brain tissues to the cerebral vasculature. The NVU consists of brain parenchymal cells including astrocytes, microglia, excitatory neurons, and inhibitory interneurons, interacting with vascular cells including pericytes (on capillaries), vascular smooth muscle cells (on arterioles and arteries), and endothelial cells. Together these cells generate an impressive filter mechanism between the blood and the brain, the blood-brain barrier (BBB)(8).
Several elements contribute to the BBB. Key features are large astrocyte end-feet, which in turn help to generate and maintain enhanced endothelial tight junctions (3), an all-encompassing basement membrane, and pericytes that have their own connections with the brain’s microglia (9). Each of these are critical to the proper function of the BBB and disruption of any are implicated in neurological diseases (10,11).
The BBB is much more than the physical barrier that we assumed in the past. It is a dynamic regulatory interface between the central nervous and immune systems. Contrary to previous ideas that the BBB protects the brain from immunological and inflammatory activities elsewhere in the body, it is now known to engage with and transport cytokines and other potentially inflammatory substances and can even allow the passage of immune cells into the CNS. The wider immune system also directly affects its performance in these processes so has a direct impact via the BBB on the health of the brain (12).
The BBB is a notable, even dominating factor in neuroinflammation in which microglia and other brain cells are activated and become disruptive. Neuroinflammation is now understood as a key basis in neurodegeneration and the onset of dementia, chronic fatigue syndromes, depressive and bipolar conditions, psychoses and a range of other challenging brain conditions.
BBB permeability and compromised performance generally increases with age, and notably with dementia of vascular origin (13).
The ‘glymphatic system’
The role of venous and fluid drainage from the brain in health and disease has only recently become apparent following advances in scanning technologies.
The brain has no lymphatic circulation and therefore cerebrospinal fluid (CSF) picks up extracellular solutes. It has been demonstrated that CSF enters the brain parenchyma alongside capillaries (in perivascular spaces formed by astroglial cells) and that brain interstitial fluid is cleared along paravenous drainage pathways (14).
The ‘glymphatic system’ is a term now used for the mechanism using these perivascular spaces to promote efficient elimination of soluble proteins and metabolites from the central nervous system. Besides waste elimination, the glymphatic system also facilitates brain-wide distribution of several compounds, including glucose, lipids, amino acids, growth factors, and neuromodulators (15).
Importantly the glymphatic system functions mainly during sleep and is largely disengaged during wakefulness. The biological need for sleep across all species may therefore reflect that the brain must enter a state of activity that enables elimination of potentially neurotoxic waste products, including β-amyloid (16).
Perivascular CSF depends on normal elastic arteriolar pulsation and becomes slower and
irregular if arteriolar pulsatility increases, such as with raised blood pressure, leading to fluid stagnation in perivascular spaces (marked by their increased visibility in MRI scans) and impaired interstitial flushing.
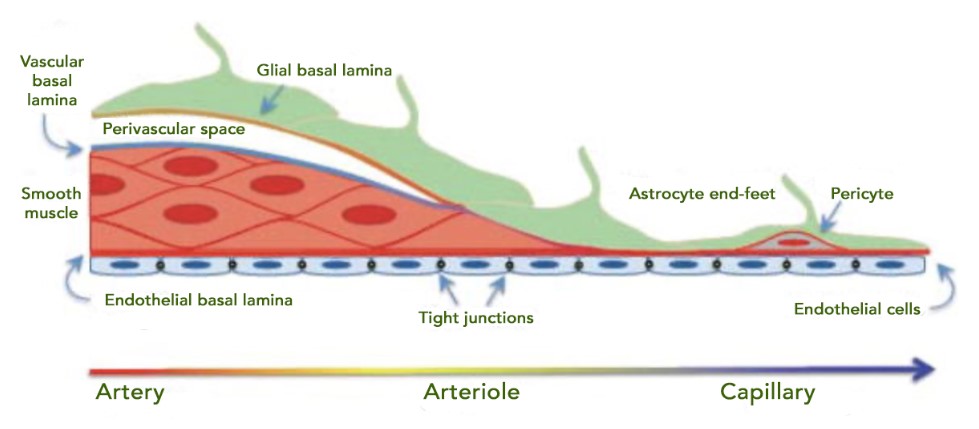
Cerebral small vessel disease
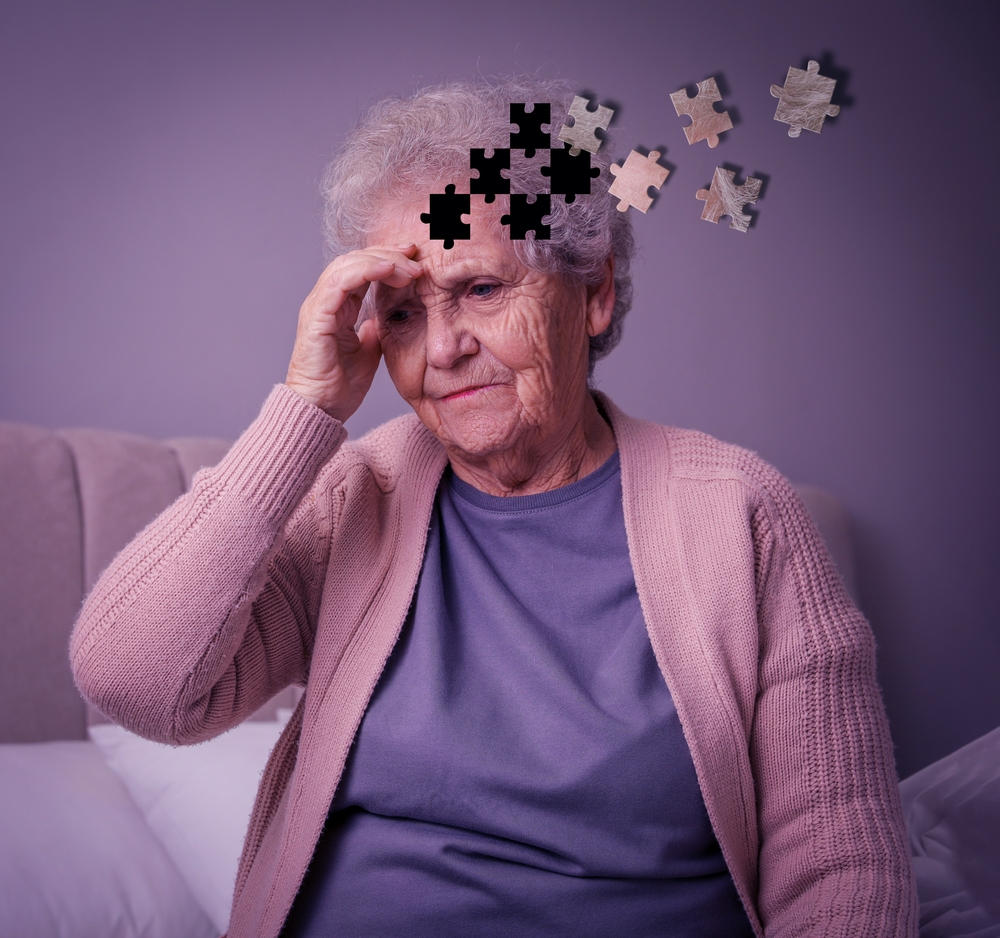
Cerebral small vessel disease (CSVD) is a broad category of disruptions to arterioles, capillaries, and venules in the CNS. It is detectable on MRI and CT scans in most haemorrhagic strokes and is also associated with cognitive impairment and dementia. Human research has demonstrated that CSVD includes several manifestations of cerebral endothelial dysfunction including impaired vasodilation, vessel stiffening, disrupted blood flow and glymphatics, and BBB damage. Direct consequences include ischaemia, neuroinflammation, myelin damage, and secondary neurodegeneration. There are various diagnoses with different impacts, e.g. cerebral amyloid angiopathy with microbleeds, or superficial siderosis with haemosiderin deposits in the superficial layers of the CNS. Key risk factors for CSVD are hypertension, smoking, diabetes, and hypercholesterolemia (17).
It is noteworthy that the impact of high blood pressure here is likely to be correctible: in a widescale autopsy study the presence of more than two microinfarcts, but no other pathological change, was independently associated with systolic BP in participants aged 65–80, though not in older participants. These associations were not seen in participants treated for hypertension (18). According to a later meta-analysis of 17 studies, patients with untreated hypertension had a significantly higher risk (42%) of developing dementia than healthy individuals. However, there was no significant difference in the risk of developing dementia between individuals with treated hypertension and healthy individuals (19). For herbal practitioners this significantly adds to the imperative of keeping blood pressure low even if that means with conventional anti-hypertensives.
A particular feature of reduced blood flow to the brain, especially with increasing age, is white matter rarefaction (leukoaraiosis) appearing as MRI ‘flares’. These involve hyperactivity of glial cells (gliosis) and demyelination following inhibited oligodendritic maturation — itself a direct consequence of endothelial dysfunction (20) and are also linked to the particularly lengthy, tortuous and thus vulnerable arteriolar vessels in the white matter (21).
MRI scans of leukoaraiosis also show a form of venous insufficiency: periventricular venous collagenosis. Cerebral veins normally thicken with age: in this case a much greater thickening is detected, resulting in narrowed lumina and even occlusion. This is likely to add to challenges in removing toxic metabolites identified with the glymphatic system also being compromised (22).
Hormesis and the consequences of impaired cerebral circulation
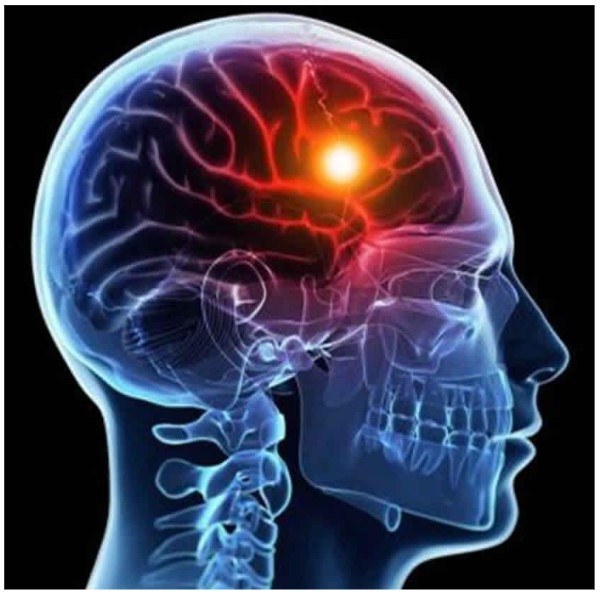
Hypoperfusion or impaired circulation deprives the brain of its two paramount trophic substances, oxygen and nutrients, and is the most critical factor in developing cognitive impairment and dementia. It triggers proinflammatory cytokines and oxidative-nitrosative stress that in turn decreases nitric oxide and enhances endothelin-1, increases amyloid-β deposition, cerebral amyloid angiopathy, further blood-brain barrier disruption, and subsequent synaptic dysfunction. These factors persist for decades, upregulating amyloid and tau before actual symptoms of the cognitive decline (23).
The brain accounts for such high levels of oxygen that this deficit (hypoxia) is particularly damaging, with additional disruptions in amyloid-β metabolism (24). Actually, hypoxia has hormetic effects (adaptive cell responses to stress) in brain health. In other words, it prompts a biphasic dose-response, with low-dose beneficial effect and a high-dose inhibitory or toxic effect. Thus, as every Victorian doctor knew when prescribing mountain air to their rich patients, reducing environmental oxygen can be healthy. Controlled hypoxia is still a treatment strategy for age-related neurological disorders. Too much oxygen is damaging and is associated with the production of reactive oxygen species (ROS), and lowering oxygen levels after the sustained generation of ROS may be helpful. Hypoxia is also a key stimulus to a protective angiogenesis in the brain: after an infarct capillary density increases within a week, with angiogenesis complete before three weeks (25). The balancing act of avoiding both O2 deprivation and toxicity has been compared to Ulysses’ perilous navigation between Scylla and Charybdis (26). Interestingly it has been reported that dietary quinones, semiquinones, phenolics, vitamins, amino acids, isoprenoids, and vasoactive compounds can down-regulate HIF-1 degradative pathways (27).
Most cellular responses to hypoxia are associated with a family of transcription factors called hypoxia-inducible factors (HIFs). HIF-1α and HIF-1β modulate the expression of genes encoding vascular endothelial growth factor (VEGF), erythropoietin and genes that are involved in glucose transport or glycolysis. In the CNS, these genes regulate neurogenesis, nerve cell differentiation, and neuronal apoptosis (28). In the brain, VEGF is a key regulator of both angiogenesis and neurogenesis, and has a neuroprotective effect in the recovery from stroke. However, it is another hormetic agent: when overactivated in acute damage it leads to increased BBB permeability and neuroinflammation (29). Because it also leads to angiogenesis in cancer, most pharmaceutical research has been into anti-VEGF agents! As we shall see below, plant polyphenols may be a modulatory factor in VEGF activity.
Yet another hormetic agency in relation to brain circulation is nitric oxide (NO). As is widely known, NO is a powerful dilator of blood vessels with antihypertensive, antithrombotic, anti-atherosclerotic and anti-obesogenic properties, and its role in healthy cerebral circulation has long been known (30,31). On the other hand, elevated production of NO, with increased nitrosative/oxidative stress, is a feature of many pathologies — such as neurodegenerative diseases, inflammation, and ischaemia (32). Again, it appears that polyphenols may have beneficial effects in modulating this activity (33).
Implications for herbal practice
Plant remedies have exciting and even unique prospects for improving brain health through their effects on its vasculature. Because of their gentle supportive activity and the convenience and inherent safety in daily dosing, they could prove to be more effective approaches for the long term than modern pharmaceuticals. For the latter, there is justification and some evidence for the use of antihypertensives, specific anti-inflammatories (such as monocycline), anti-platelet factors and statins. However, the evidence for all of these is at best mixed (34). The plant prospects touched on below include many that can be taken daily as lifestyle contributions without the need for constant herbal prescriptions.
The evidence touched on above, increasingly definitive as new research is published, is that we can see the brain and its challenges as at least as a vascular as much as a neurological matter. It is now certain that the brain tissue and its functions are accessible through the vasculature and that practitioners should now look to circulatory remedies for maintaining its healthy function, including in neurological, neurodegenerative and even mental and psychological disorders.
Also check additional promise for plants that can modulate neuroinflammation in our article “Neuroinflammation: An emerging role for herbs and spices?“.
Promising plant principles
Many dietary components such as curcumin, resveratrol, blueberry polyphenols, sulforaphane, polyunsaturated fatty acids (PUFAs), and diets enriched with polyphenols and PUFAs have been shown to induce neurogenesis in adult brains (35,36).
There is evidence to suggest that flavonoids and other shikimate derivatives may be capable of preventing many forms of cerebrovascular disease, including those associated with stroke and dementia, with powerful evidence for the beneficial effects of flavonoids on endothelial function and peripheral blood flow (37).
Polyphenols also:
- Enhance the production of vasodilating factors: nitric oxide (NO), endothelium-derived hyperpolarizing factor (EDHF) and prostacyclin;
- Inhibit the synthesis of vasoconstrictor endothelin-1 in endothelial cells; and
- Inhibit the over expression of two major pro-angiogenic factors, VEGF and MMP-2 in smooth muscle cells (38).
One proposition is that flavonols (notably metabolites of (-)-catechin from green tea) increase endothelial NO levels with reduction in NADPH oxidase activity. A moderate level of endothelial NADPH oxidase is essential for angiogenesis and wound healing, but in another example of hormesis (see above) risks endothelial dysfunction when upregulated by proinflammatory agonists. Dietary polyphenols may contribute to maintain safe NADPH oxidase activity (39).
Flavonoids demonstrate direct benefits on neural mitochondria and neuroglobin activity (40,41). Polydatin, genistein, daidzein, biochanin A, and formononetin upregulate neuroglobin (Ngb) promoter activity, increasing Ngb mRN expression in primary neurons. The flavonoid naringenin and the stilbene resveratrol up-regulate Ngb levels and increase its levels in mitochondria of human neuroblastoma cells (42).
However, many polyphenols do not reach the CNS, and it will be worth considering indirect ways in which they could influence brain structure and function (43). Could the effects follow productive crosstalk in the gut microbiome? Polyphenols interact with intestinal microbiota to activate short-chain fatty acid production, intestinal immune function, and other protective physiological processes (44). This mechanism has been cited as perhaps the key to their impressive properties: “This interplay provides the central explanation as to the stupefying pleiotropic biological/clinical actions and functions of ‘parent’ polyphenols even though their systemic bioavailability is incredibly limited” (45). The microbiome metabolises non-absorbable polyphenol molecules to more available simple phenolics. Quercetin is ‘C-ring cleaved’ to 3,4-dihydroxyphenylacetic acid (DOPAC) and 3-hydroxyphenylacetic acid (OPAC) which strongly inhibit the formation of advanced glycation end-products and oxidative stress-induced neuronal cell death (46).
Herbs and spices to watch
The following plants already show promise, and in many cases persuasive evidence, in supporting brain health via vascular mechanisms. Note that most of these can be considered dietary ingredients and are thus very suitable for lifestyle recommendations. This almost certainly reflects the weight of research interest in foodstuffs: one can confidently predict that many ingredients from the herbal dispensary would be shown to have similar properties. The practitioner can be encouraged to look with renewed interest on any remedy currently used for its circulatory effects with renewed interest as potential ‘new nervines’!
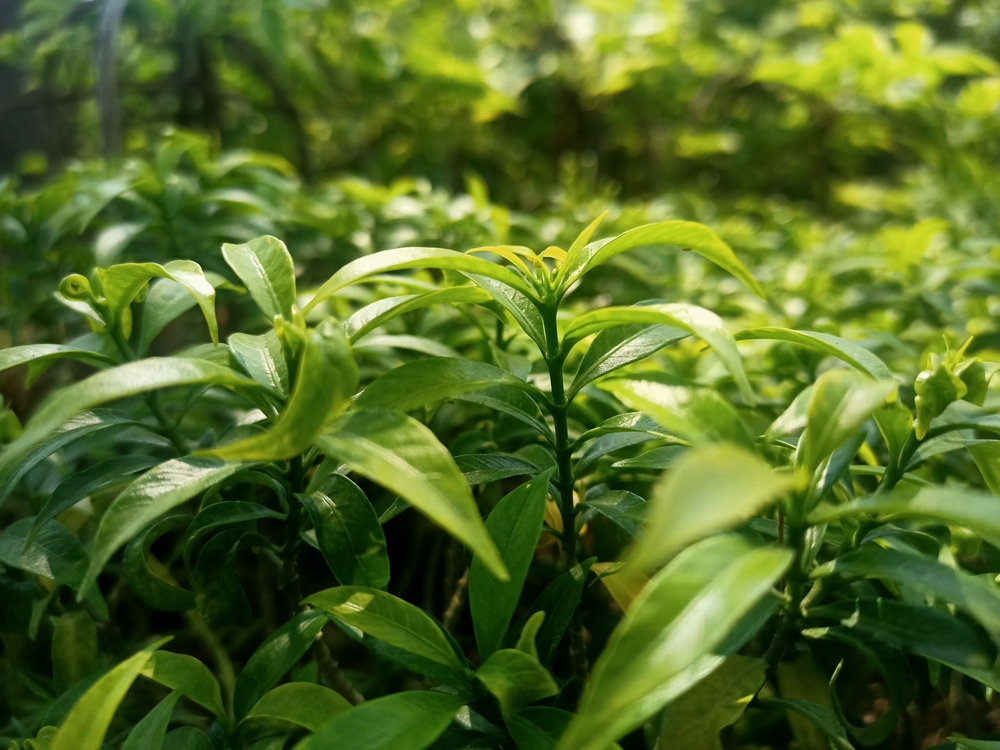
Tea
Tea polyphenols such as epigallocatechin gallate or EGCG are readily absorbed, cross the blood-brain barrier, and can be detected in the brain (47). A number of studies have shown that they have neuroprotective and neurogenerative effects (48,49,50,51). They are also likely to be metabolised by gut microbiota into metabolites (e.g. EGCG into g-valerolactones) themselves with significant neuroprotective effects (52), or may work otherwise indirectly by restoring dysbiosis (53).
Cocoa
Cocoa contains high quantities of flavonals catechins and epicatechins (even more of thee than tea), along with theobromine, an alkaloid with vasodilator properties. Cognitive benefit has been seen in human trials and are likely to derive from improved vascular function and neurovascular coupling that result in better cerebral blood flow, insulin resistance, blood pressure, interactions with signalling pathways linked to neurogenesis and neuroprotection, and reductions in neural inflammation (54).
Grapes (and resveratrol)
Resveratrol has confirmed NO-mediated vasodilatory and endothelial protective activity (55).
Studies suggest that resveratrol may improve cognitive performance through direct benefits to the BBB (56) including in the face of systemic infection (57). Grape polyphenols other than resveratrol may also exert neurological health benefits (58).
Soy
Soya isoflavone daidzein is metabolised by gut microbiota to S-equol: the most anti-atherogenic of isoflavones. The evidence associates both atherosclerosis and arterial stiffness with cognitive decline and dementia, and points to the benefit of S-equol especially in preventing these (59).
Spices
Culinary spices pack more phytochemical power (and circulatory stimulation!) than other plants and their consumption is widely associated with neuroprotective benefits (60). They include the following examples.
Ginger
Constituents with cholinesterase-inhibiting and neuroprotective properties have been shown to cross the BBB. Preliminary studies show improvements in cognitive functions, including a dose-dependent effect in a placebo-controlled study of a standardised ginger extract (61,62).
Turmeric
A range of studies shows oral curcumin consumption improved cognitive performance and increased neurogenerative activity (63,64,65). Benefits may not rely only on the bioavailability of curcumin: curcumin and its metabolites may act indirectly on the CNS by correcting dysbiosis of gut microbiome to influence the microbiota-gut-brain axis, and is subject to bacterial enzymatic modifications, forming pharmacologically more active metabolites than curcumin (66,67) There is also evidence for the benefits of ar-tumerone (68).
Cayenne
Several studies have shown the neuroprotective role of capsaicin in neurological disorders (69). Cayenne has long been recognised as the most effective circulatory stimulant and vasodilator (70). One factor may be its confirmed action on transient receptor potential vanilloid 1 (TRPV1) with evidence that this modulates neuroinflammatory processes (71).
Cinnamon
Multiple activities across metabolic and vascular functions have been identified, with a particular prospect for improving cognitive performance and neuroprotection through reducing insulin resistance, e.g. in prediabetics (72,73).
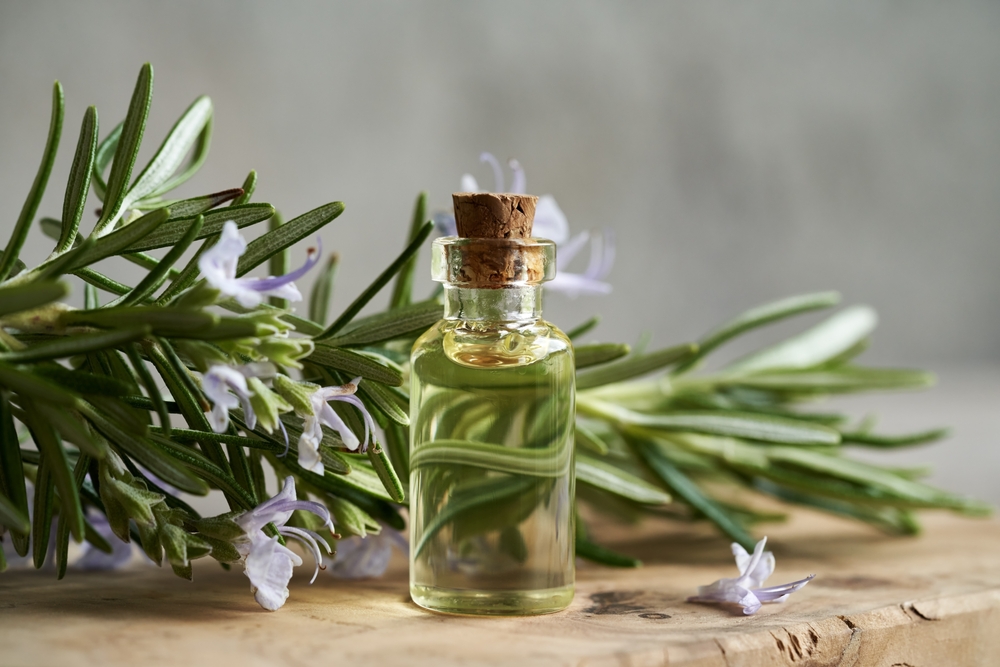
Rosemary
“There’s rosemary; that’s for remembrance” — Shakespeare
Rosemary has demonstrated anticholinesterase activity, enhanced Nrf2 processes and endothelial modulation (74). Its diterpene carnosic acid exerted protective effects upon neuronal cells more intensely than resveratrol or sulforaphane (75). Our own clinical trial pointed to rosemary improving cognition in the elderly (76).
Sage
“Sage will retard the rapid progress of decay that treads upon our heels so fast in latter years of life, will preserve faculty and memory more valuable to the rational mind than life itself.” — John Hill, Family Herbal, 18th century
Sage extracts also exhibit cholinesterase inhibition, with 1 μL essential oils being more potent than the recommended daily dose of donepezil (an aceltylcholinesterase inhibitor used to treat dementia). This was attributed to the presence of di- and triterpenes (77).
Ginkgo
The standardised 50:1 extract EGb761 is the paramount circulatory prescription in German medicine, often used in recovery from stroke, in vascular tinnitus and other heart and circulatory disorders. It is also used widely in psychiatric medicine. It is very rich in flavonoids (recently showing BBB relevant activities of their own (78)) as well as unique ginkgolides. EGb761 at 240 mg/day is able to stabilise or slow decline in cognition, function, behaviour, and global change at 22–26 weeks in cognitive impairment and dementia, especially for patients with neuropsychiatric symptoms (79).
References
- Porkert M. The Theoretical Foundations of Chinese Medicine: Systems of Correspondence. MIT Press; 1974.
- Vogenstahl J, et al. Vascular Regulation of Developmental Neurogenesis. Front Cell Dev Biol. 2022;10:890852. doi:10.3389/fcell.2022.890852
- Bautch VL, James JM. Neurovascular development: The beginning of a beautiful friendship. Cell Adh Migr. 2009;3(2):199-204. doi:10.4161/cam.3.2.8397. PMID:19363295
- Tabata H. Crosstalk between Blood Vessels and Glia during the Central Nervous System Development. Life (Basel). 2022;12(11):1761. doi:10.3390/life12111761. PMID:36362915
- Ruhrberg C, Bautch VL. Neurovascular development and links to disease. Cell Mol Life Sci. 2013;70(10):1675-1684. doi:10.1007/s00018-013-1277-5. PMID:23475065
- Rattner A, Wang Y, Nathans J. Signaling Pathways in Neurovascular Development. Annu Rev Neurosci. 2022;45:87-108. doi:10.1146/annurev-neuro-111020-102127. PMID:35803586
- Franklin RJM, ffrench-Constant C. Regenerating CNS myelin – from mechanisms to experimental medicines. Nat Rev Neurosci. 2017;18(12):753-769. doi:10.1038/nrn.2017.136. PMID:29142295
- Ward NL, Lamanna JC. The neurovascular unit and its growth factors: coordinated response in the vascular and nervous systems. Neurol Res. 2004;26(8):870-883. doi:10.1179/016164104X3798. PMID:15727271
- Morris GP, Foster CG, Courtney JM, et al. Microglia directly associate with pericytes in the central nervous system. Glia. 2023;71(8):1847-1869. doi:10.1002/glia.24371. PMID:36994950
- Cheng J, Korte N, Nortley R, et al. Targeting pericytes for therapeutic approaches to neurological disorders. Acta Neuropathol. 2018;136:507-523. doi:10.1007/s00401-018-1893-0
- Brown LS, Foster CG, Courtney JM, et al. Pericytes and Neurovascular Function in the Healthy and Diseased Brain. Front Cell Neurosci. 2019;13:282. doi:10.3389/fncel.2019.00282. PMID:31316352
- Erickson MA, Dohi K, Banks WA. Neuroinflammation: a common pathway in CNS diseases as mediated at the blood-brain barrier. Neuroimmunomodulation. 2012;19(2):121-130. doi:10.1159/000330247. PMID:22248728
- Farrall AJ, Wardlaw JM. Blood-brain barrier: ageing and microvascular disease – systematic review and meta-analysis. Neurobiol Aging. 2009;30(3):337-352. doi:10.1016/j.neurobiolaging.2007.07.015
- Iliff JJ, et al. A paravascular pathway facilitates CSF flow through the brain parenchyma and the clearance of interstitial solutes, including amyloid β. Sci Transl Med. 2012;4(147):147ra111. doi:10.1126/scitranslmed.3003748
- Hablitz LM, Nedergaard M. The Glymphatic System: A Novel Component of Fundamental Neurobiology. J Neurosci. 2021;41(37):7698-7711. doi:10.1523/JNEUROSCI.0619-21.2021. PMID:34526407
- Chong PLH, Garic D, Shen MD, et al. Sleep, cerebrospinal fluid, and the glymphatic system: A systematic review. Sleep Med Rev. 2022;61:101572. doi:10.1016/j.smrv.2021.101572. PMID:34902819
- Wardlaw JM, Smith C, Dichgans M. Small vessel disease: mechanisms and clinical implications. Lancet Neurol. 2019;18(7):684-696. doi:10.1016/S1474-4422(19)30079-1. PMID:31097385
- Wang LY, et al. Blood pressure and brain injury in older adults: findings from a community-based autopsy study. J Am Geriatr Soc. 2009;57(11):1975-1981. doi:10.1111/j.1532-5415.2009.02493.x.
- Lennon MJ, Lam BCP, Lipnicki DM, et al. Use of Antihypertensives, blood pressure, and estimated risk of dementia in late life: an individual participant data meta-analysis. JAMA Netw Open. 2023;6(9):e2333353. doi:10.1001/jamanetworkopen.2023.33353.
- Rajani RM, Williams A. Endothelial cell-oligodendrocyte interactions in small vessel disease and aging. Clin Sci (Lond). 2017;131(5):369-379. doi:10.1042/CS20160618. PMID:28202749
- Brown WR, Thore CR. Cerebral microvascular pathology in ageing and neurodegeneration. Neuropathol Appl Neurobiol. 2011;37(1):56-74. doi:10.1111/j.1365-2990.2010.01139.x
- Brown WR, Thore CR. Cerebral microvascular pathology in ageing and neurodegeneration. Neuropathol Appl Neurobiol. 2011; 37(1): 56-74. doi: 10.1111/j.1365-2990.2010.01139.x
- Daulatzai MA. Cerebral hypoperfusion and glucose hypometabolism: Key pathophysiological modulators promote neurodegeneration, cognitive impairment, and Alzheimer’s disease. J Neurosci Res. 2017;95(4):943-972. doi: 10.1002/jnr.23777
- Peers C, Dallas ML, Boycott HE, et al. Hypoxia and neurodegeneration. Ann N Y Acad Sci. 2009;1177:169-177. doi: 10.1111/j.1749-6632.2009.05026.x. PMID: 19845619
- Yang XT, Bi YY, Feng DF. From the vascular microenvironment to neurogenesis. Brain Res Bull. 2011;84(1):1-7. doi: 10.1016/j.brainresbull.2010.09.008. PMID: 20850508
- Burtscher J, Mallet RT, Burtscher M, Millet GP. Hypoxia and brain aging: Neurodegeneration or neuroprotection? Ageing Res Rev. 2021;68:101343. doi: 10.1016/j.arr.2021.101343. PMID: 33862277
- Losso JN, Bawadi HA. Hypoxia inducible factor pathways as targets for functional foods. J Agric Food Chem. 2005;53(10):3751-3768. doi: 10.1021/jf0479719.
- Mitroshina EV, Savyuk MO, Ponimaskin E, Vedunova MV. Hypoxia-Inducible Factor (HIF) in Ischemic Stroke and Neurodegenerative Disease. Front Cell Dev Biol. 2021;9:703084. doi: 10.3389/fcell.2021.703084. PMID: 34395432
- Geiseler SJ, Morland C. The Janus Face of VEGF in Stroke. Int J Mol Sci. 2018;19(5):1362. doi: 10.3390/ijms19051362
- Lee TJ. Nitric oxide and the cerebral vascular function. J Biomed Sci. 2000;7(1):16-26. doi: 10.1007/BF02255914. PMID: 10644885
- Tewari D, Sah AN, Bawari S, et al. Role of Nitric Oxide in Neurodegeneration: Function, Regulation, and Inhibition. Curr Neuropharmacol. 2021;19(2):114-126. doi: 10.2174/1570159X18666200429001549. PMID: 32348225; PMCID: PMC8033982
- Liu C, Liang MC, Soong TW. Nitric Oxide, Iron and Neurodegeneration. Front Neurosci. 2019;13:114. doi: 10.3389/fnins.2019.00114. PMID: 30833886
- Serreli G, Deiana M. Role of Dietary Polyphenols in the Activity and Expression of Nitric Oxide Synthases: A Review. Antioxidants (Basel). 2023;12(1):147. doi: 10.3390/antiox12010147. PMID: 36671009
- Gao Y, Li D, Lin J, et al. Cerebral small vessel disease: Pathological mechanisms and potential therapeutic targets. Front Aging Neurosci. 2022;14:961661. doi: 10.3389/fnagi.2022.961661. PMID: 36034144
- Poulose SM, Miller MG, Shukitt-Hale B. Nutritional Factors Affecting Adult Neurogenesis and Cognitive Function. Adv Nutr. 2017;8(6):804-811. doi: 10.3945/an.117.016261
- Lewis JE, Melillo AB, Tiozzo E, Chen L, Leonard S. The effects of twenty-one nutrients and phytonutrients on cognitive function: A narrative review. J Clin Transl Res. 2021;7(4):575-620. PMID: 34541370
- Vauzour D, Vafeiadou K, Rodriguez-Mateos A, Rendeiro C, Spencer JPE. The neuroprotective potential of flavonoids: a multiplicity of effects. Genes Nutr. 2008;3(3-4):115-126. doi: 10.1007/s12263-008-0091-4
- Stoclet JC, Chataigneau T, Ndiaye M, et al. Vascular protection by dietary polyphenols. Eur J Pharmacol. 2004;500(1-3):299-313. doi: 10.1016/j.ejphar.2004.07.034
- Schewe T, Steffen Y, Sies H. How do dietary flavanols improve vascular function? A position paper. Arch Biochem Biophys. 2008;476(2):102-106. doi: 10.1016/j.abb.2008.03.004
- Fiocchetti M, Nuzzo D, Totta P, et al. Neuroglobin and mitochondria: The impact on neurodegenerative diseases. Arch Biochem Biophys. 2021;701:108823. doi: 10.1016/j.abb.2021.108823
- Schaffer S, et al. Effects of polyphenols on brain ageing and Alzheimer’s disease: focus on mitochondria. Mol Neurobiol. 2012;46(1):161-178. doi:10.1007/s12035-012-8282-9.
- Sandoval-Acuña C, et al. Polyphenols and mitochondria: an update on their increasingly emerging ROS-scavenging independent actions. Arch Biochem Biophys. 2014;559:75-90. doi:10.1016/j.abb.2014.05.017.
- Schaffer S, Halliwell B. Do polyphenols enter the brain and does it matter? Some theoretical and practical considerations. Genes Nutr. 2012;7:99-109. doi:10.1007/s12263-011-0255-5.
- Kawabata K, et al. Role of Intestinal Microbiota in the Bioavailability and Physiological Functions of Dietary Polyphenols. Molecules. 2019;24(2):370. doi:10.3390/molecules24020370.
- Koudoufio M, et al. Insight into Polyphenol and Gut Microbiota Crosstalk: Are Their Metabolites the Key to Understand Protective Effects against Metabolic Disorders? Antioxidants (Basel). 2020;9(10):982. doi:10.3390/antiox9100982.
- Murota K, et al. Flavonoid metabolism: the interaction of metabolites and gut microbiota. Biosci Biotechnol Biochem. 2018;82(4):600-610. doi:10.1080/09168451.2018.1444467.
- Haskell-Ramsay CF, Schmitt J, Actis-Goretta L. The Impact of Epicatechin on Human Cognition: The Role of Cerebral Blood Flow. Nutrients. 2018;10(8):986. doi:10.3390/nu10080986. PMID:30060538.
- Unno K, Nakamura Y. Green Tea Suppresses Brain Aging. Molecules. 2021;26(16):4897. doi:10.3390/molecules26164897. PMID:34443485.
- Lee SB, Choi EH, Jeong KH, et al. Effect of catechins and high-temperature-processed green tea extract on scavenging reactive oxygen species and preventing Aβ1-42 fibrils’ formation in brain microvascular endothelium. Nutr Neurosci. 2020;23(5):363-373. doi:10.1080/1028415X.2018.1507618. PMID:30111271.
- Chen G, Cheng K, Niu Y, et al. (-)-Epicatechin gallate prevents inflammatory response in hypoxia-activated microglia and cerebral edema by inhibiting NF-κB signaling. Arch Biochem Biophys. 2022;729:109393. doi:10.1016/j.abb.2022.109393. PMID:36084697.
- Chen T, Yang Y, Zhu S, et al. Inhibition of Aβ aggregates in Alzheimer’s disease by epigallocatechin and epicatechin-3-gallate from green tea. Bioorg Chem. 2020;105:104382. doi:10.1016/j.bioorg.2020.104382. PMID:33137558.
- Zhang Z, Zhang Y, Li J, et al. The Neuroprotective Effect of Tea Polyphenols on the Regulation of Intestinal Flora. Molecules. 2021;26(12):3692. doi:10.3390/molecules26123692. PMID:34204244.
- Murota K, et al. Flavonoid metabolism: the interaction of metabolites and gut microbiota. Biosci Biotechnol Biochem. 2018;82(4):600-610. doi:10.1080/09168451.2018.1444467.
- Baker LD, Manson JE, Rapp SR, et al. Effects of cocoa extract and a multivitamin on cognitive function: A randomized clinical trial. Alzheimers Dement. 2023;19(4):1308-1319. doi:10.1002/alz.12767. PMID:36102337.
- Xia N, Förstermann U, Li H. Resveratrol and endothelial nitric oxide. Molecules. 2014;19(10):16102-16121. doi:10.3390/molecules191016102. PMID:25302702.
- Katila N, Duwa R, Bhurtel S, et al. Enhancement of blood-brain barrier penetration and the neuroprotective effect of resveratrol. J Control Release. 2022;346:1-19. doi:10.1016/j.jconrel.2022.04.003. PMID:35398173.
- Yang R, Lv Y, Miao L, et al. Resveratrol Attenuates Meningitic Escherichia coli-Mediated Blood-Brain Barrier Disruption. ACS Infect Dis. 2021;7(4):777-789. doi:10.1021/acsinfecdis.0c00564. PMID:33723986.
- Pasinetti GM, et al. Roles of resveratrol and other grape-derived polyphenols in Alzheimer’s disease prevention and treatment. Biochim Biophys Acta. 2015;1852(6):1202-1208. doi:10.1016/j.bbadis.2014.10.006.
- Sekikawa A, Ihara M, Lopez O, et al. Effect of S-equol and Soy Isoflavones on Heart and Brain. Curr Cardiol Rev. 2019;15(2):114-135. doi:10.2174/1573403X15666181205104717. PMID:30516108.
- Kannappan R, Gupta SC, Kim JH, et al. Neuroprotection by spice-derived nutraceuticals: you are what you eat! Mol Neurobiol. 2011;44(2):142-159. doi:10.1007/s12035-011-8168-2. PMID:21360003.
- Arcusa R, Villaño D, Marhuenda J, et al. Potential Role of Ginger (Zingiber officinale Roscoe) in the Prevention of Neurodegenerative Diseases. Front Nutr. 2022;9:809621. doi:10.3389/fnut.2022.809621. PMID:35369082.
- Schepici G, Contestabile V, Valeri A, Mazzon E. Ginger, a Possible Candidate for the Treatment of Dementias? Molecules. 2021;26(18):5700. doi:10.3390/molecules26185700. PMID:34577171.
- Sarker MR, Franks SF. Efficacy of curcumin for age-associated cognitive decline: a narrative review of preclinical and clinical studies. Geroscience. 2018;40(2):73-95. doi:10.1007/s11357-018-0017-z.
- Berry A, Collacchi B, Masella R, Varì R, Cirulli F. Curcuma Longa, the “Golden Spice” to Counteract Neuroinflammaging and Cognitive Decline-What Have We Learned and What Needs to Be Done. Nutrients. 2021;13(5):1519. doi:10.3390/nu13051519. PMID:33946356.
- Voulgaropoulou SD, van Amelsvoort TAMJ, Prickaerts J, Vingerhoets C. The effect of curcumin on cognition in Alzheimer’s disease and healthy aging: A systematic review of pre-clinical and clinical studies. Brain Res. 2019;1725:146476. doi:10.1016/j.brainres.2019.146476. PMID:31560864.
- Di Meo F, Margarucci S, Galderisi U, et al. Curcumin, Gut Microbiota, and Neuroprotection. Nutrients. 2019;11(10):2426. doi:10.3390/nu11102426. PMID:31614630.
- Lopresti AL. The Problem of Curcumin and Its Bioavailability: Could Its Gastrointestinal Influence Contribute to Its Overall Health-Enhancing Effects? Adv Nutr. 2018;9(1):41-50. doi:10.1093/advances/nmx011.
- Hucklenbroich J, Klein R, Neumaier B, et al. Aromatic-turmerone induces neural stem cell proliferation in vitro and in vivo. Stem Cell Res Ther. 2014;5(4):100. doi:10.1186/scrt500. PMID:25928248.
- Tyagi S, Shekhar N, Thakur AK. Protective Role of Capsaicin in Neurological Disorders: An Overview. Neurochem Res. 2022;47(6):1513-1531. doi:10.1007/s11064-022-03549-5. PMID:35150419.
- Yang D, Luo Z, Ma S, et al. Activation of TRPV1 by dietary capsaicin improves endothelium-dependent vasorelaxation and prevents hypertension. Cell Metab. 2010;12(2):130-141. doi:10.1016/j.cmet.2010.05.015. PMID:20674858.
- Kong WL, Peng YY, Peng BW. Modulation of neuroinflammation: Role and therapeutic potential of TRPV1 in the neuro-immune axis. Brain Behav Immun. 2017;64:354-366. doi:10.1016/j.bbi.2017.03.007. PMID:28342781.
- Yulug B, Cankaya S. Translational perspective: is cinnamon a suitable agent for cognitive impairment and Alzheimer’s disease associated with brain trauma? Neural Regen Res. 2019;14(8):1372-1373. doi:10.4103/1673-5374.253518.
- Wahlqvist ML, Lee MS, Lee JT, et al. Cinnamon users with prediabetes have a better fasting working memory: a cross-sectional function study. Nutr Res. 2016;36(4):305-310. doi:10.1016/j.nutres.2015.12.005. PMID:27001275.
- Habtemariam S. The Therapeutic Potential of Rosemary (Rosmarinus officinalis) Diterpenes for Alzheimer’s Disease. Evid Based Complement Alternat Med. 2016;2016:2680409. doi:10.1155/2016/2680409.
- de Oliveira MR. The Dietary Components Carnosic Acid and Carnosol as Neuroprotective Agents: a Mechanistic View. Mol Neurobiol. 2016;53(9):6155-6168. doi:10.1007/s12035-015-9519-1.
- Pengelly A, Snow J, Mills SY, et al. Short-term study on the effects of rosemary on cognitive function in an elderly population. J Med Food. 2012;15(1):10-17. doi:10.1089/jmf.2011.0005. PMID:21877951.
- Kennedy DO, Scholey AB. The psychopharmacology of European herbs with cognition-enhancing properties. Curr Pharm Des. 2006;12(35):4613-4623. doi:10.2174/138161206779010387.
- Hu WH, Wang HY, Xia YT, et al. Kaempferol, a Major Flavonoid in Ginkgo Folium, Potentiates Angiogenic Functions in Cultured Endothelial Cells by Binding to Vascular Endothelial Growth Factor. Front Pharmacol. 2020;11:526. doi:10.3389/fphar.2020.00526. PMID:32410995.
- Tan MS, et al. Efficacy and adverse effects of ginkgo biloba for cognitive impairment and dementia: a systematic review and meta-analysis. J Alzheimer’s Dis. 2015;43(2):589-603. doi:10.3233/JAD-140837.