Robert Verkerk discusses key factors associated with metabolic disease and important treatment approaches.
Under the skin of the metabolic disease crisis
The metabolic disease crisis afflicts a large part of the global population, particularly those in higher-income and emerging industrialised countries, and represents the single largest preventable, non-communicable disease burden in these countries (1). The covid-19 pandemic has shone a spotlight on how a single viral pathogen can exploit comorbidities that are the result of metabolic dysfunction, creating havoc in its wake. It is surely also a reminder of the urgent need to address the underlying metabolic crisis, especially among younger members of society who will otherwise become extremely vulnerable both to chronic and infectious diseases.
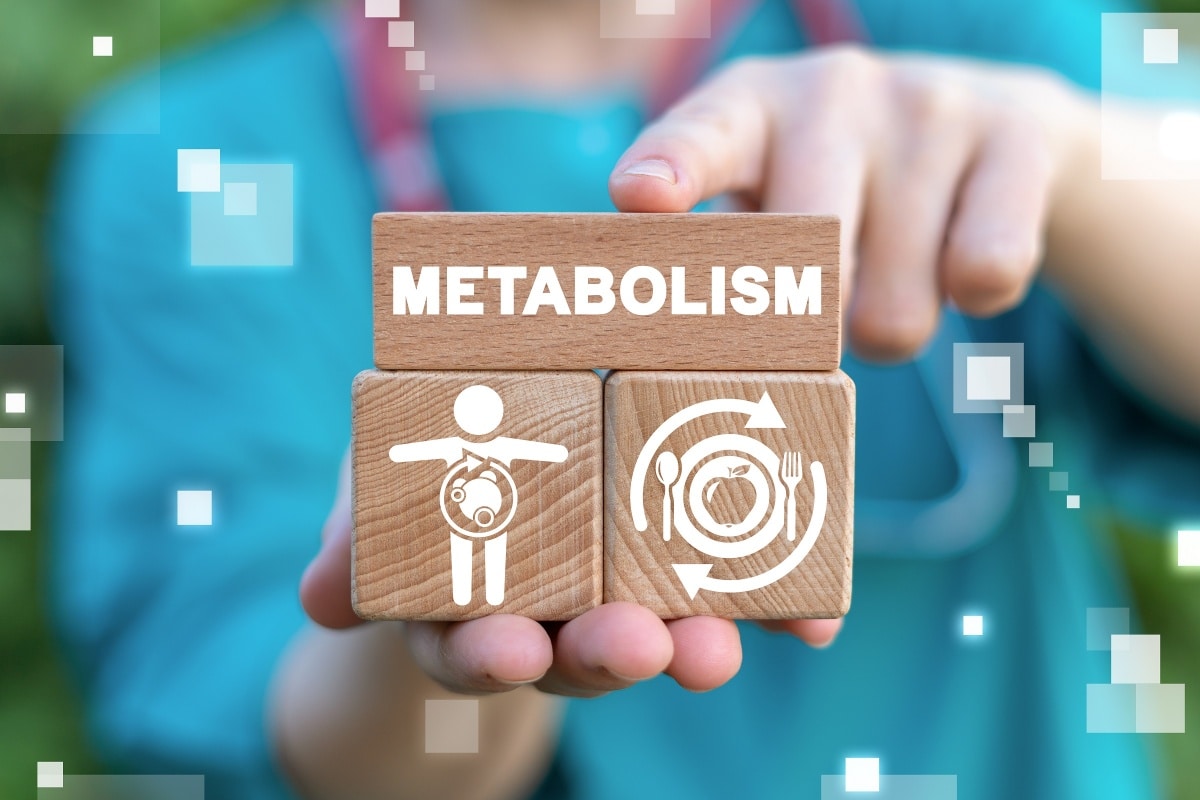
Obesity, type 2 diabetes and ischaemic heart disease are three of the most pronounced downstream manifestations of the metabolic crisis. However, increasingly, many types of cancer are considered to have a metabolic basis (2), while the rapid rise in prevalence of Alzheimer’s and dementia in industrialised countries is also thought to be, at least partially, mediated by metabolic disturbance, hence the increasingly common reference to type 3 diabetes (3).
There is overwhelming evidence that the causes are both variable, between individuals, yet are also multi-factorial, being a consequence of our poor adaptation to modern lifestyle and dietary patterns.
The Global Burden of Disease Study (2015) (4) identified four metabolic risk factors that are widely associated with the dysregulated metabolism that is typically associated with diet and lifestyle mediated metabolic diseases, these being:
- Raised systolic blood pressure
- Overweight and especially obesity
- Hyperglycemia (high fasting blood glucose levels), and
- Hyperlipidemia (high levels of fat in the blood).
These physiological risk factors are in turn associated with other physiological factors, as well as a broad array of social, environmental and genetic contributory factors. Given huge differences in the response both by individuals and different population groups to these multiple determinants of metabolic disease, there is little scientific consensus over which are the most important combination of factors for particularly diseases or population groups.
This uncertainty is compounded by the common lack of clarity around the causality of non-communicable and metabolic diseases, that is much harder to ascertain than is the case of infectious (communicable) diseases.
Key factors associated with metabolic disease
Among the plethora of factors associated with the increased or reduced risk of metabolic diseases that contribute to many of the leading causes of preventable and premature deaths, notably ischemic heart disease, stroke, some cancers (breast, prostate, colorectal), obesity, type 2 diabetes and low bone mineral density, are the following:
- Dysregulated energy and glucose metabolism (5)
- Excessive and overly-frequent intake of sugars and refined carbohydrates, in association with reduced fibre intake, that contribute to chronically raised blood sugar (hyperglycaemia) and subsequently insulin resistance (6)
- Dysregulated lipid metabolism, in particular the inability to beta-oxidise (burn) fatty acids in adipose tissue and the development of non-alcoholic fatty liver (7)
- Dysregulated ‘cross-talk’ between adipocytes in adipose tissue (subcutaneous fat), macrophages (immune system) and neurons (nervous system) (8)
- Hormonal dysregulation of appetite, a complex process involving multiple hormones including grehlin (hunger), leptin (satiation) and adiponectin (fat burning). Apart from involving the endocrine system, it also involves the gut and its microbiome, the brain, the central and autonomic nervous systems, the immune system, adipose tissue and a large number of signalling compounds and nutrient-sensing systems such mTOR and AMPK (9)
- Low bone mineral density caused by disturbances to metabolism of calcium, other minerals and low circulating vitamin D status (10)
- Low grade, chronic systemic inflammation and oxidative stress (11)
- Chronic stress and inadequate quantity and quality of sleep (12)
- Exposure to air pollution and environmental toxins (13)
- The bidirectional relationship between physical inactivity and obesity (14)
- The prevalence of obesogenic environments and ‘anthropogens’ (human-made environments, their by-products and/or lifestyles encouraged by these, some of which may be detrimental to human health) (15)
- Social determinants of metabolic disease including socio-economic status, structural and cultural factors (16)
- The interplay of genetics and epigenetics in gene expression (17)
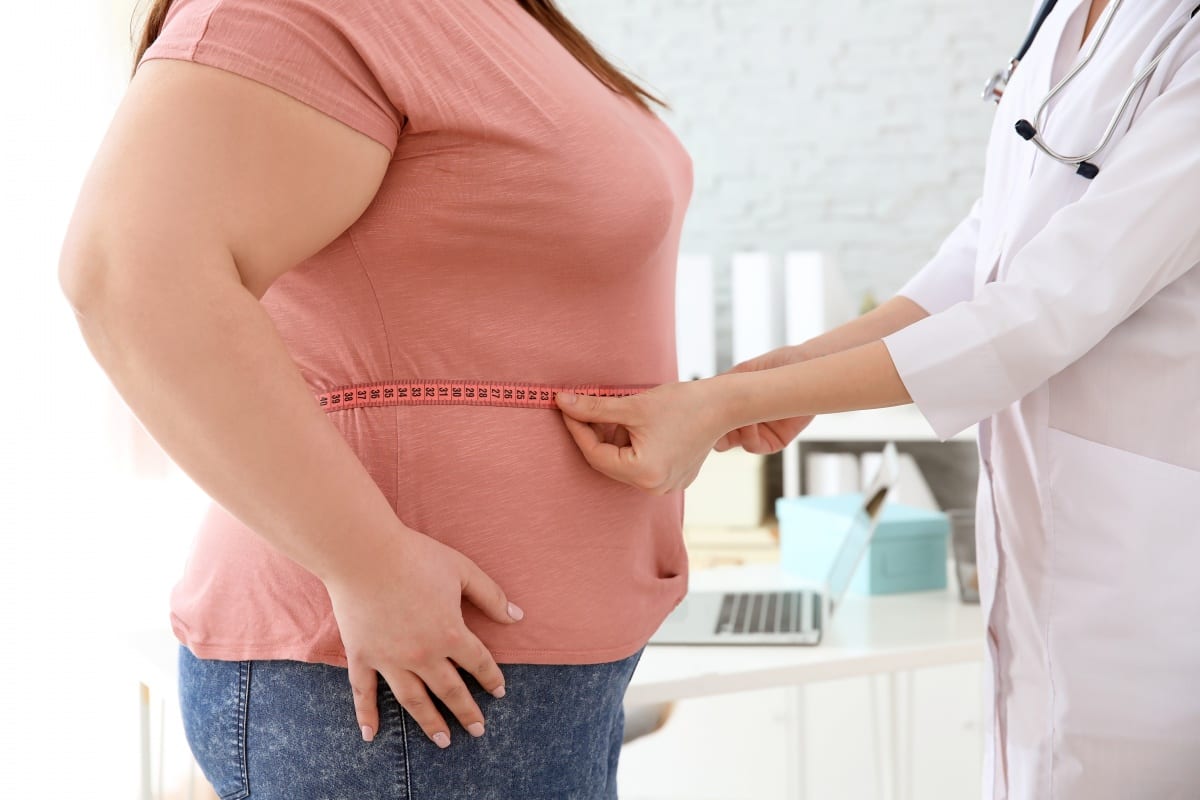
Not only are these associated factors both multiple and highly variable, very few have been identified as direct, causal factors. Several years ago, when metabolically-triggered low-grade systemic inflammation (sometimes referred to as ‘metainflammation’) was linked to obesity and chronic diseases like type 2 diabetes and ischaemic heart disease, there was hope that resolving inflammation alone would resolve these two great burdens of disease (18). More recently it has been found that metainflammation may or may not be associated with obesity while it is consistently connected with a range of lifestyle-related and environmental factors such as diet, inactivity, smoking, sleep, chronic stress and pollution exposure (19).
Leading Australian obesity and metabolic disease clinicians and researchers, Drs Garry Egger and John Dixon, accordingly suggested that “obesity may often be just an accomplice to, as much as a perpetrator of, many metabolic diseases” and that solutions should be directed to trying to reduce citizen exposure to anthropogens and obesogenic environments (20).
The same authors also provide a framework that can be used to help better understand the hierarchy of determinants. These can be classified as ‘downstream’ (i.e. cause), ‘midstream’ (i.e. cause of the cause) or ‘upstream’ (i.e. cause of the cause of the cause) (Figure 1).
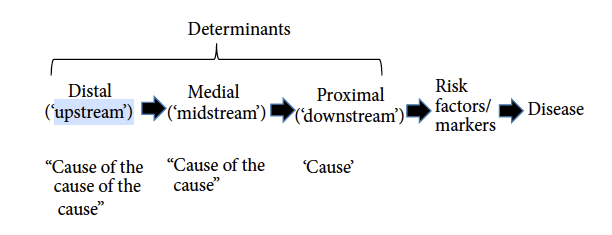
Eggers & Dixon also tabulate, using the acronym NASTIE ODOURS, a wide range of determinants (Box 1) of chronic disease along with evidence of how specific factors may increase or decrease risk, or act as moderators (mediators).
BOX 1 – Lifestyle & environmental determinants (‘anthropogens’) of metabolic disease
N = Nutrition (lack of diversity, inadequate plant foods/phytonutrients, poor eating patterns, excess intake of refined carbohydrates
A = In(Activity) (e.g. sedentary behaviour/work, lack of stretching/dynamic movement)
S = Stress, anxiety and depression
T = Technology-induced pathology (e.g. screens, machinery, highly processed foods)
I = Inadequate sleep (quantity and quality)
E = Environment (political/economic, recreation and green spaces, pollution)
O = Occupation (e.g. social justics, work equality, security)
D = Drugs, smoking and alcohol
O and U = Over- and Under-exposure (e.g. sunlight, radiation, asbestos)
R = Relationships (e.g. companionship, peer support, love)
S = Social factors (e.g. poverty socio-economic status, education, security)
Adapted from Eggers & Dixon (2014) (20)
Systems approaches, not silver bullets
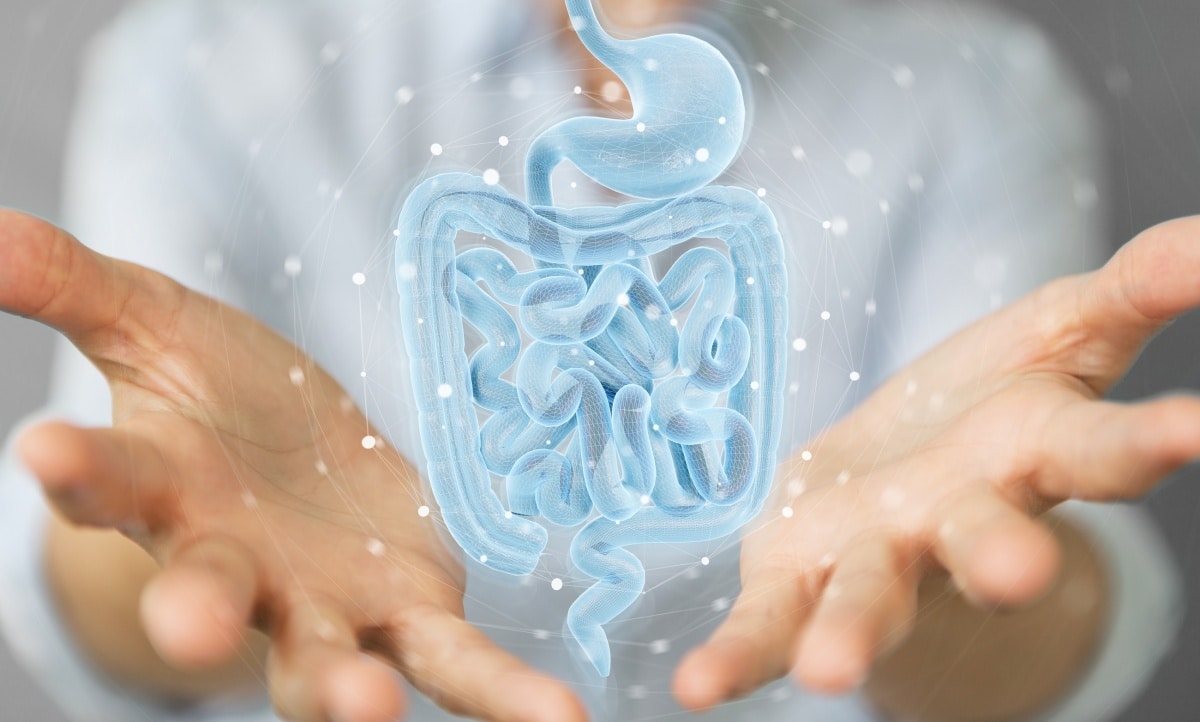
Despite the obvious difficulties in modifying some of the determinants of metabolic disease, such as underlying genetic predisposition or an individual’s place of residence or work, there are a very large number of factors that can be altered in ways that can, sometimes dramatically, improve health outcomes. Even an individual’s genetic predisposition can be altered by changing the inner and outer environment to which the individual is exposed, which in turn changes the pattern of gene expression (i.e. epigenetics) (21).
Four over-arching priorities are identified below. These are open to modification, optimal outcomes often being realised when interventions or protocols are personalised, following guidance by the individual’s health practitioner, around the specific needs, capacities and environments experienced by the individual.
For the purposes of this article, examples of possible interventions are drawn mainly from the fields of nutrition and herbal medicine. These interventions are of particular value given the multi-target, and often, multi-system actions, of nutrients and synergistic plant compounds.
1. Restoring blood sugar metabolism and metabolic flexibility
Metabolic flexibility refers to the adaptive ability to have “a clear capacity to utilize lipid and carbohydrate fuels and to transition between them.” (22) When this capacity is lost, i.e. metabolic inflexibility occurs, an individual is usually primarily reliant on burning carbohydrates to produce adenosine triphosphate (ATP) via the mitochondria. This inflexibility is associated with ‘metabolic syndrome’ that afflicts obese and type 2 diabetic individuals which is characterised by: 1) excessive insulin response to elevated blood sugar; 2) a failure of skeletal muscle tissue to transition from using fatty acids (lipid) as an energy source during the fasting state and carbohydrate in the insulin-stimulated prandial state, and; 3) a tendency to store excess energy as fat (23).
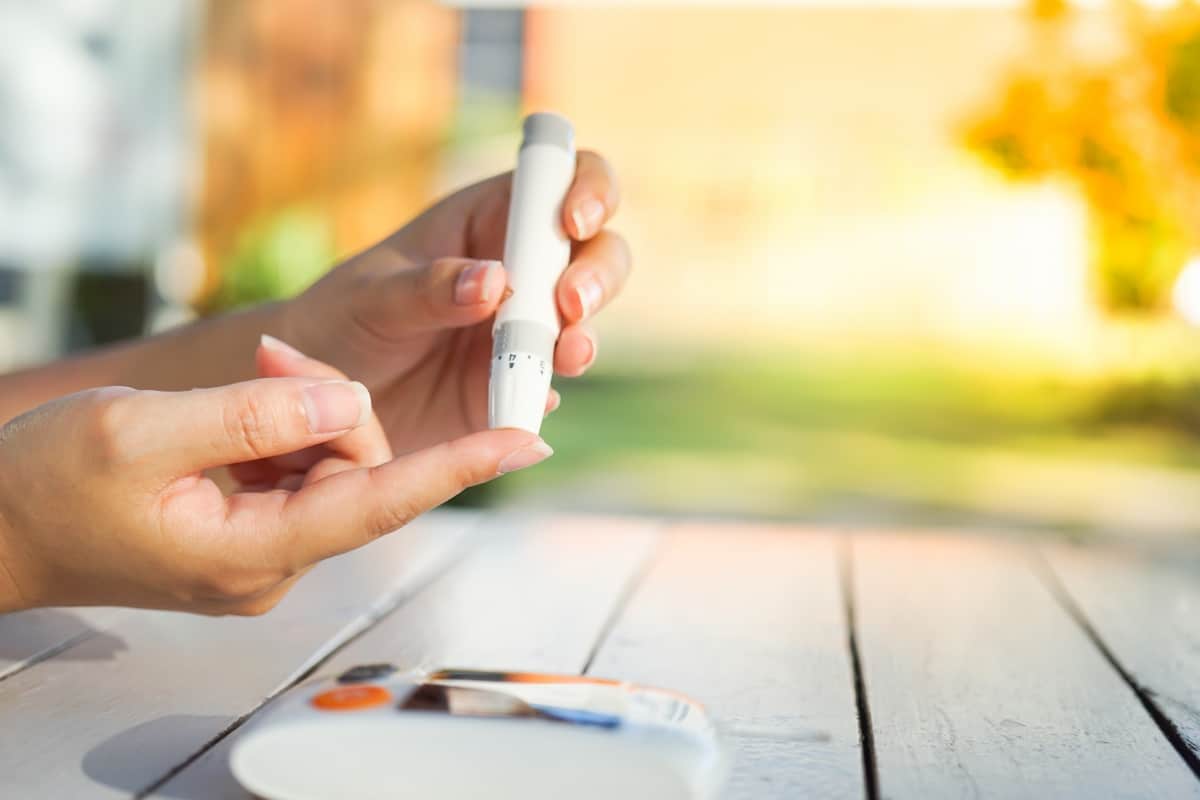
Blood sugar spikes can be greatly reduced by eliminating or reducing consumption of sugars, refined carbohydrates (e.g. bread, rice, pasta), as well as starchy vegetables. Moreover, extending the fasting intervals (e.g. at least 5 hours between eating intervals, 12-16 hour overnight fasts, or 8-hour time-restricted feeding) can help to re-educate the neuro-endocrine system to beta-oxidise fatty acids. This adaptive process, that may take several weeks or even months, culminates in the liver-generating ketone bodies that can be used as an energy source, the process being referred to as ‘keto-adaptation’ (24). It is always non-existent in cases of non-alcoholic fatty liver disease (25).
Extremely helpful in supporting this process of keto-adaptation are herbal products that are able to blunt blood sugar responses following carbohydrate-containing meals. In this regard cinnamon has been intensively studied and used clinically, often used both as an infusion in tea or in powdered form in capsules. Not only has it been found to be able to reduce post-prandial blood-glucose spikes, it can also increase insulin sensitivity and reduce fat accumulation in the liver (26,27,28). Trial data tends to be highly variable, this likely being the result of different profiles and concentrations of key bioactive constituents, such as chalcones, flavonoids, tannins and coumarins, these also varying between Cinnamomum species. Commonly used species include Cinnamomum zeylanicum (Ceylon cinnamon), aka Cinnamomum verum (true cinnamon), and Cinnamomum cassia (Saigon cinnamon), the latter often containing coumarins which may, at high intake levels, be of concern for those with variations of the CYP2A6 gene that impact coumarin metabolism (29).
With a wealth of historical use and trial data behind it, is triphala, the Ayurvedic combination of three fruits, namely amla (Emblica officanalis), haritaki (Terminalia chebula)and bibhitaki (Terminalia bellirica). The formula has been shown to act as a powerful antioxidant, anti-inflammatory and blood-sugar regulator (30). Another widely used combination is fruit extracts from haritaki, and the oleoresin of guggul (Commiphora wightii [formerly C. mukul]) and myrrh (Commiphora myrrha), widely available in a daily preparation of 200 mg of each (31).
2. Facilitating physical activity and mitochondrial biogenesis
Public health messaging often advises citizens to exercise more or be more active without underlying consideration of their metabolism and physiology. Many people who are inactive have sub-optimal energy yielding pathways and benefit from cofactors that facilitate function. These include a full array of bioactive, naturally-occurring forms of B vitamins as well as coenzyme Q10, all of these being cofactors in mitochondrial respiration via the Kreb’s cycle.
In addition to these micronutrients, research is increasingly pointing to an ever more diverse array of phytonutrients facilitating mitochondrial function and ATP generation. These phytonutrients are generally absent or very limited in simplified Western, processed food-based diets and an array were reviewed by Tao et al (2019) (32), as follows:
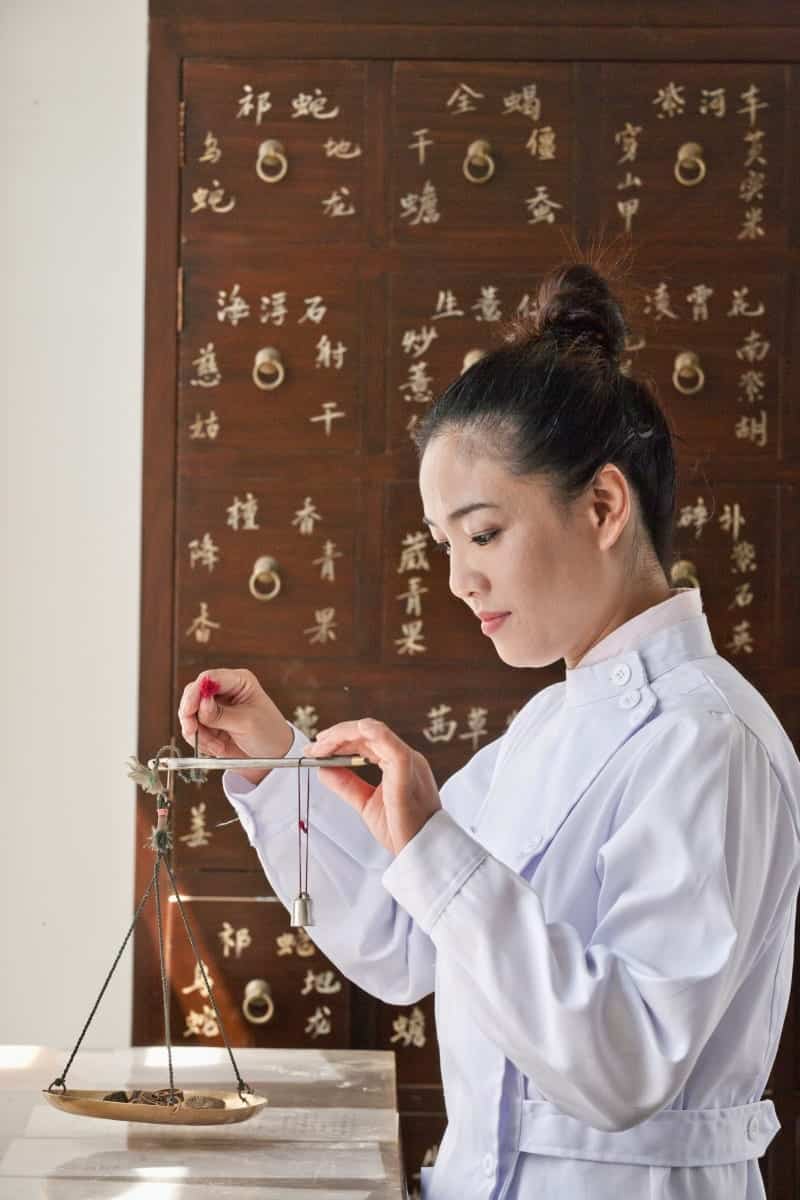
- Homoisoflavanone-1: a natural compound in the Chinese herbal medicine, Polygonatum odoratum
- Myricetin: a flavonol compound found in multiple herbs
- Esculetin: a natural coumarin compound found in traditional medicinal herbs
- Curcumin: the principle component of Curcuma longa rhizome/root
- [6]-Gingerol: the key bioactive component of ginger
- Triptolide: a natural product from Tripterygium wilfordii
- Thymoquinone: an active constituent of Nigella sativa seeds
- Epigallocatechin gallate (EGCG): a polyphenol from green tea
- Artepillin C: a bioactive component of Brazilian green propolis
- Allicin: predominant component of freshly crushed garlic
- Ganoderma atrum polysaccharide
- Ginsenosides: a major pharmacologically active ingredients of ginseng
- Jolkinolide B: a diterpenoid from Euphorbia fischeriana
- Withaferin A: a steroidal lactone of Withania somnifera (ashwagandha)
- Cucurbitacin B: a bioactive compound from Pedicellus melo.
3. Anti-inflammatory dietary patterns
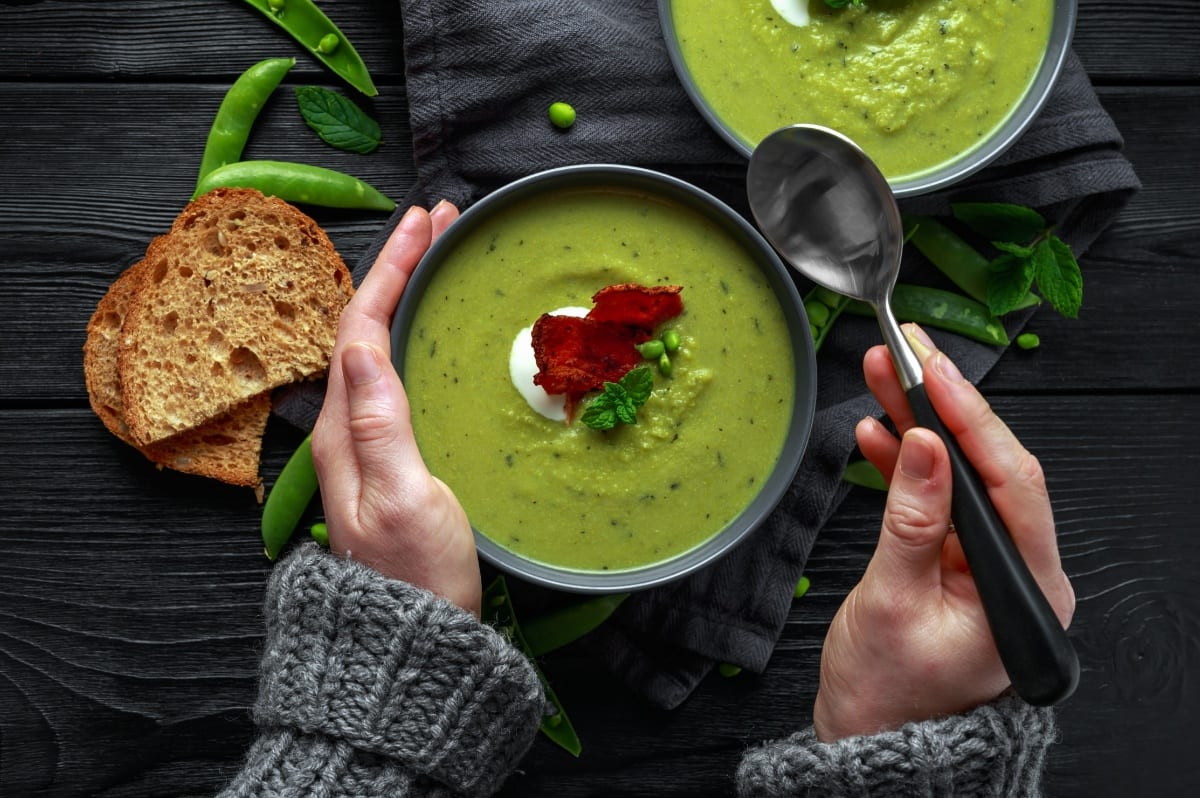
There is increasing recognition in the scientific community that food serves much more than being a source of energy or calories for the body. Rather is provides the body with information – and critical to this is the diversity of nutrients provided by diverse, nutrient-dense diets, along with culinary and medicinal herbs. Furthermore, evidence is accumulating that suggests nutrition in the first 1000 days of lives is an important contributor to long-term healthspan (33).
Nutrient-density and dietary diversity have been shown to relate directly to the anti-inflammatory nature of diets (34).
Anti-inflammatory diets have been widely studied and tend to be plant-based, rich in polyphenols and fibre, while being lower in omega-6 fatty acids, sugars and refined or processed foods compared with conventional diets (35).
Given that every eating event triggers an inflammatory response in the body, anti-inflammatory dietary patterns are also generally associated with intermittent fasting or time-restricted feeding.
4. Stress transformation and sleep quality
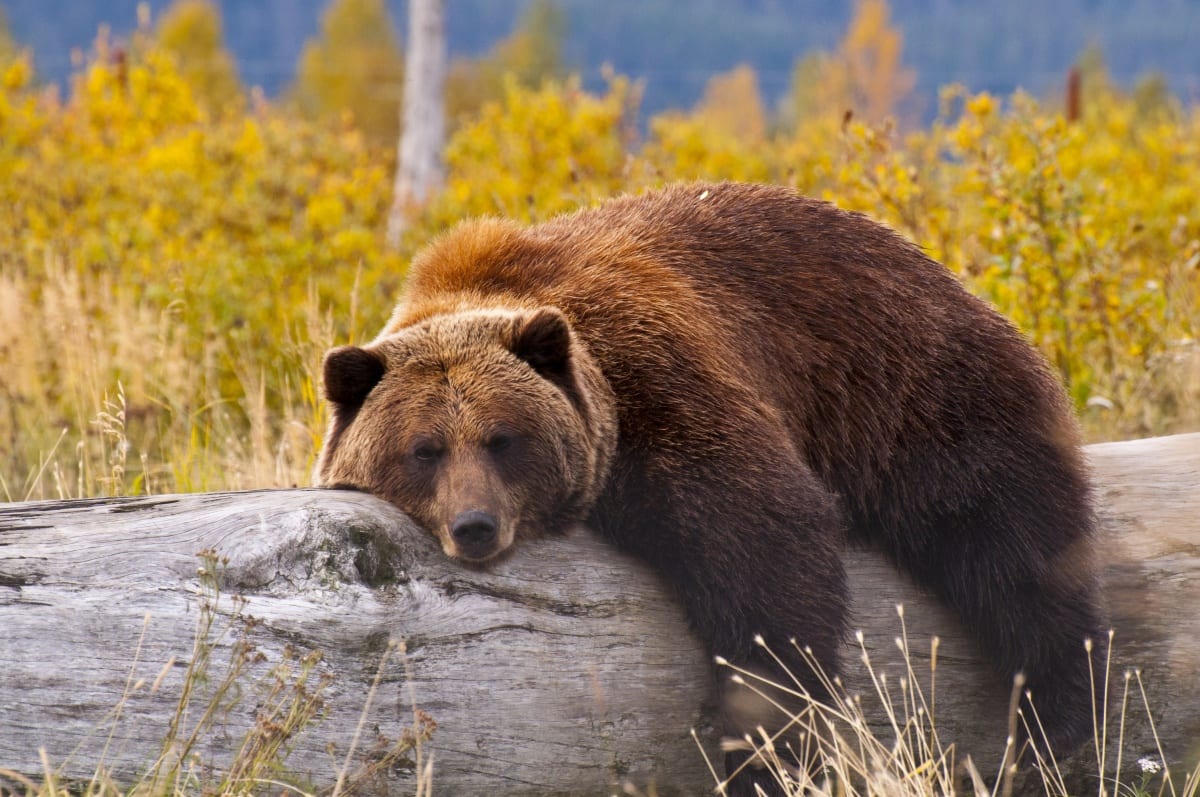
The damaging effects of stress are caused by the response to stress, rather than the stressor itself. Stress-modulating herbs, especially adaptogens (36), have been shown to be invaluable to help improve the stress response under conditions of persistent, chronic stress that are all too common in modern day lives.
Well studied and clinically-proven adaptogens include:
- Rhodiola rosea, root (37)
- Withania somnifera (ashwagandha), root (38)
- Panax quniquefolius, root (39)
- Schisandra chinensis, fruits, seeds (39)
- Eleutherococcus senticosus (Siberian ginseng), root (36)
Research since the 1980s has revealed ever more about the critical role of the endocannabinoid system (ECS) in maintaining balanced mood and a resilient stress response (40). This includes evidence of the deleterious role of chronic, psychoemotional stress on cannabinoid receptors in the brain and central nervous system (41). This provides good clinical justification for use of high quality, full spectrum CBD-standardised that cannabinoid receptors to help modulate the response of cannabinoid receptors in those exposed to such ongoing chronic stressors (42).
Additionally, several herbal medicines have been conclusively demonstrated to facilitate sleep duration and quality. These include:
- Valeriana officinalis (valerian) (43,44)
- Passiflora incarnata (passionflower) (45)
- Melissa officinalis (lemon balm) (44)
- Eschscholzia californica (Californian poppy) (44)
- Lavandula angustifolia (lavender) (44)
Conclusions
It is clear that top-down public health measures have had little impact on curtailing the spiral of metabolic disease that is running rampant through the majority of the industrialised world. The impact caused by SARS-CoV-2 is a reminder of how pathogens can interact with unhealthy, imbalanced body systems and cause severe disease and societal disruption.
While it may seem that proven solutions to the metabolic crisis are still wanting, there is copious clinical evidence of successes in reversing metabolic diseases, although little of this is adequately documented or published in peer reviewed journals. Adding to this difficulty is that the disease-centric nature of prevailing medical systems means that very little effort is expended studying dietary and lifestyle patterns associated with the healthiest populations, the research on the world’s ‘blue zones’ of exceptional longevity being an exception (46).
Self-care and practitioner-guided self-care, the democratisation and even digitisation of health are key elements of any solution to the crisis, as recognised by the Lancet Global Health Commission of 2018 (47) and others.
Central to this will be multi-factorial, whole system-focused approaches and interventions that include at their heart the diversification of natural, plant-based diets. Additionally, such strategies should include increased intake of sustainably produced phytonutrients and herbal medicines which are increasingly being shown to be capable of restoring homeostatic balance in those suffering the effects of metabolic disturbance or dysfunction.
References
- World Health Organization. Global status report on noncommunicable diseases 2014. WHO, Geneva [http://apps.who.int/iris/bitstream/handle/10665/148114/9789241564854_eng.pdf;jsessionid=A42614E96691C5441534CE0A0F440F51?sequence=1]
- Seyfried TN, Flores RE, Poff AM, et al. Cancer as a metabolic disease: implications for novel therapeutics, Carcinogenesis 2014; 35(3): 515–527. [Link: https://academic.oup.com/carcin/article/35/3/515/2463440?login=true]
- de la Monte SM, Wands JR. Alzheimer’s Disease is Type 3 Diabetes—Evidence Reviewed. Journal of Diabetes Science and Technology. 2008;2(6):1101-1113. [Link: https://journals.sagepub.com/doi/abs/10.1177/193229680800200619].
- GBD 2015 Risk Factors Collaborators. Global, regional, and national comparative risk assessment of 79 behavioural, environmental and occupational, and metabolic risks or clusters of risks, 1990–2015: a systematic analysis for the Global Burden of Disease Study 2015. Lancet, 2016; 388(10053): 1659-1724. [Link: https://www.thelancet.com/journals/lancet/article/PIIS0140-6736(16)31679-8/fulltext]
- Timper K, Brüning JC. Hypothalamic circuits regulating appetite and energy homeostasis: pathways to obesity. Dis Model Mech. 2017; 10(6): 679-689. [Link: https://www.ncbi.nlm.nih.gov/pmc/articles/PMC5483000/]
- Gross LS, Li L, Ford ES, et al. Increased consumption of refined carbohydrates and the epidemic of type 2 diabetes in the United States: an ecologic assessment. Am J Clin Nutr, 2004; 79(5): 774–779. [Link: https://academic.oup.com/ajcn/article/79/5/774/4690186?login=true]
- Leamy AK, Egnatchik RA, Young JD. Molecular mechanisms and the role of saturated fatty acids in the progression of non-alcoholic fatty liver disease. Progr Lipid Res, 2013; 52(1): 165-174. [Link: https://www.sciencedirect.com/science/article/abs/pii/S0163782712000586]
- Boura-Halfon S, Pecht T, Jung S, et al. Obesity and dysregulated central and peripheral macrophage-neuron cross-talk. Eur J Immunol. 2019; 49(1): 19-29. [Link: https://pubmed.ncbi.nlm.nih.gov/30407631/]
- Hotamisligil G, Erbay E. Nutrient sensing and inflammation in metabolic diseases. Nat Rev Immunol, 2008; 8: 923–934.
- Gennari C. Calcium and vitamin D nutrition and bone disease of the elderly. Public Health Nutr 2001; 4: 547-559. [Link: https://www.cambridge.org/core/journals/public-health-nutrition/article/calcium-and-vitamin-d-nutrition-and-bone-disease-of-the-elderly/B2522D7574073DEBF8B6E051E8D9A63A]
- Frühbeck G, Catalán V, Rodríguez A et al. Involvement of the leptin-adiponectin axis in inflammation and oxidative stress in the metabolic syndrome. Sci Rep 7, 2017; 6619. [Link: https://www.nature.com/articles/s41598-017-06997-0#citeas]
- Mullington JM, Haack M, Toth M, et al. Cardiovascular, inflammatory and metabolic consequences of sleep deprivation. Prog Cardiovasc Dis. 2009; 51(4): 294–302. [Link: https://www.ncbi.nlm.nih.gov/pmc/articles/PMC3403737/]
- Vrijheid M, Fossati S, Maitre L, et al. Early-life environmental exposures and childhood obesity: an exposome-wide approach. Environ Health Perspect. 2020;128(6):67009. doi:10.1289/EHP5975. [https://www.ncbi.nlm.nih.gov/pmc/articles/PMC7313401/]
- Myers A, Gibbons C, Finlayson G, et al. Associations among sedentary and active behaviours, body fat and appetite dysregulation: investigating the myth of physical inactivity and obesity. Brit J Sports Med 2017; 51: 1540-1544.[Link: https://bjsm.bmj.com/content/51/21/1540]
- Egger G, Dixon J. Beyond obesity and lifestyle: a review of 21st century chronic disease determinants. Biomed Res Int. 2014; 2014: 731685. [Link: https://pubmed.ncbi.nlm.nih.gov/24804239/]
- Cockerham WC, Hamby BW, Oates GR. The social determinants of chronic disease. Am J Prev Med. 2017; 52(1S1): S5-S12. [Link: https://www.ncbi.nlm.nih.gov/pmc/articles/PMC5328595/]
- Diels, S, Vanden Berghe, W, Van Hul, W. Insights into the multifactorial causation of obesity by integrated genetic and epigenetic analysis. Obes Rev. 2020; 21: e13019. [Link: https://onlinelibrary.wiley.com/doi/abs/10.1111/obr.13019]
- Hotamisligil, GS. Inflammation and metabolic disease. Nature 2006; 444: 860–867. [Link: https://www.nature.com/articles/nature05485?report=reader]
- Medzhitov R. Origin and physiological roles of inflammation. Nature. 2008; 454(7203): 428-35. [Link: https://pubmed.ncbi.nlm.nih.gov/18650913/]
- Egger G, Dixon J (2009). Obesity and chronic disease: Always offender or often just accomplice? Brit J Nutr, 2009; 102(8): 1238-1242. [Link: https://www.cambridge.org/core/journals/british-journal-of-nutrition/article/obesity-and-chronic-disease-always-offender-or-often-just-accomplice/C106E532E8D623D5AB4F5D60B0D38DF8].
- Abdul QA, Yu BP, Chung HY, et al. Epigenetic modifications of gene expression by lifestyle and environment. Arch. Pharm. Res. 2017; 40: 1219–1237. [Link: https://link.springer.com/article/10.1007/s12272-017-0973-3#citeas]
- Kelley DE, He J, Menshikova EV, Ritov VB. Dysfunction of mitochondria in human skeletal muscle in type 2 diabetes. Diabetes. 2002; 51: 2944–2950.[Link: https://diabetes.diabetesjournals.org/content/51/10/2944.short].
- Storlien L, Oakes ND, Kelley DE. Metabolic flexibility. Proc Nutr Soc, 2007; 63(2): 363-368. [Link: https://www.cambridge.org/core/journals/proceedings-of-the-nutrition-society/article/metabolic-flexibility/6649F3D8E24414FD6997DBFEAE8A090E]
- Freese J, Klement RJ, Ruiz-Núñez B, et al. The sedentary (r)evolution: Have we lost our metabolic flexibility? Version 2. F1000Res. 2017; 6: 1787. [Link: https://www.ncbi.nlm.nih.gov/pmc/articles/PMC5710317/]
- Shao M, Ye Z, Qin Y, et al. Abnormal metabolic processes involved in the pathogenesis of non-alcoholic fatty liver disease (Review). Exp Ther Med. 2020; 20(5): 26. [Link: https://www.ncbi.nlm.nih.gov/pmc/articles/PMC7471863/]
- Allen RW, Schwartzman E, Baker WL, et al. Cinnamon use in type 2 diabetes: an updated systematic review and meta-analysis. Ann Fam Med. 2013; 11(5): 452-9. [Link: https://pubmed.ncbi.nlm.nih.gov/24019277/]
- Deyno S, Eneyew K, Seyfe S, et al. Efficacy and safety of cinnamon in type 2 diabetes mellitus and pre-diabetes patients: A meta-analysis and meta-regression. Diabetes Res Clin Pract. 2019; 156: 107815. [Link: https://pubmed.ncbi.nlm.nih.gov/31425768/]
- Kanuri G, Weber S, Volynets V, et al. Cinnamon extract protects against acute alcohol-induced liver steatosis in mice. J Nutr. 2009; 139(3): 482-7. [Link: https://pubmed.ncbi.nlm.nih.gov/19126670/]
- Uno T, Obe Y, Ogura C, et al. Metabolism of 7-ethoxycoumarin, safrole, flavanone and hydroxyflavanone by cytochrome P450 2A6 variants. Biopharm Drug Dispos. 2013; 34(2): 87-97. [https://pubmed.ncbi.nlm.nih.gov/23112005/]
- Zhang Y, Xiang R, Fang S, et al. Experimental Study on the Effect of Tibetan Medicine Triphala on the Proliferation and Apoptosis of Pancreatic Islet β Cells through Incretin-cAMP Signaling Pathway. Biol Pharm Bull. 2020 Feb 1;43(2):289-295. [Link: https://pubmed.ncbi.nlm.nih.gov/31813891/]
- Shokoohi R, Kianbakht S, Faramarzi M, et al. Effects of an Herbal Combination on Glycemic Control and Lipid Profile in Diabetic Women: A Randomized, Double-Blind, Placebo-Controlled Clinical Trial. J Evid Based Complementary Altern Med. 2017 Oct;22(4):798-804. [Link: https://pubmed.ncbi.nlm.nih.gov/29228822/]
- Tao F, Zhang Y, Zhang Z. The role of herbal bioactive components in mitochondria function and cancer therapy. Evid Based Complement Alternat Med. 2019; 2019: 3868354. [Link: https://pubmed.ncbi.nlm.nih.gov/31308852/]
- Mameli C, Mazzantini S, Zuccotti GV. Nutrition in the First 1000 Days: The Origin of Childhood Obesity. Int J Environ Res Public Health. 2016; 13(9): 838. [Link: https://pubmed.ncbi.nlm.nih.gov/27563917/]
- Shivappa N, Steck SE, Hurley TG, et al. Designing and developing a literature-derived, population-based dietary inflammatory index. Public Health Nutr. 2014; 17(8): 1689-96. [Link: https://pubmed.ncbi.nlm.nih.gov/23941862/]
- Sears B. Anti-inflammatory diets. J Am Coll Nutr. 2015; 34 Suppl 1: 14-21. [Link: https://pubmed.ncbi.nlm.nih.gov/26400429/]
- Panossian AG, Efferth T, Shikov AN, et al. Evolution of the adaptogenic concept from traditional use to medical systems: Pharmacology of stress- and aging-related diseases. Med Res Rev. 2021; 41(1): 630-703. [Link: https://www.ncbi.nlm.nih.gov/pmc/articles/PMC7756641/]
- Anghelescu IG, Edwards D, Seifritz E, et al. Stress management and the role of Rhodiola rosea: a review. Int J Psychiatry Clin Pract. 2018; 22(4): 242-252. [Link: https://pubmed.ncbi.nlm.nih.gov/29325481/]
- Tandon N, Yadav SS. Safety and clinical effectiveness of Withania somnifera (Linn.) Dunal root in human ailments. J Ethnopharmacol. 2020; 255: 112768. [Link: https://pubmed.ncbi.nlm.nih.gov/32201301/]
- Liao LY, He YF, Li L, et al. A preliminary review of studies on adaptogens: comparison of their bioactivity in TCM with that of ginseng-like herbs used worldwide. Chin Med. 2018; 13: 57. [Link: https://www.ncbi.nlm.nih.gov/pmc/articles/PMC6240259/]
- Donvito G, Nass SR, Wilkerson JL, et al. The Endogenous Cannabinoid System: A Budding Source of Targets for Treating Inflammatory and Neuropathic Pain. Neuropsychopharmacol. 2018 43(1): 52–79.
- Rossi S, De Chiara V, Musella A, et al. Chronic psychoemotional stress impairs cannabinoid-receptor-mediated control of GABA transmission in the striatum. J Neurosci. 2008; 28(29): 7284-7292. [Link: https://www.jneurosci.org/content/28/29/7284.short]
- Scherma M, Muntoni AL, Riedel G, Fratta W, Fadda P. Cannabinoids and their therapeutic applications in mental disorders. Dialogues Clin Neurosci. 2020; 22(3): 271-279. [Link: https://www.ncbi.nlm.nih.gov/pmc/articles/PMC7605020/]
- Shinjyo N, Waddell G, Green J. Valerian root in treating sleep problems and associated disorders-a systematic review and meta-analysis. J Evid Based Integr Med. 2020; 25: 2515690X20967323. [Link: https://www.ncbi.nlm.nih.gov/pmc/articles/PMC7585905/]
- Bruni O, Ferini-Strambi L, Giacomoni E, et al. Herbal remedies and their possible effect on the GABAergic system and sleep. Nutrients. 2021; 13(2): 530. [Link: https://www.ncbi.nlm.nih.gov/pmc/articles/PMC7914492/]
- Poulain M, Herm A, Pes G. The Blue Zones: areas of exceptional longevity around the world. Vienna Yearbook Pop Res. 2013; 11: 87-108. [Link: https://www.jstor.org/stable/43050798?seq=1]
- Kruk ME, Gage AD, Arsenault C, et al. High-quality health systems in the Sustainable Development Goals era: time for a revolution. Lancet Glob Health. 2018 Nov;6(11):e1196-e1252. [Link: https://pubmed.ncbi.nlm.nih.gov/30196093/]