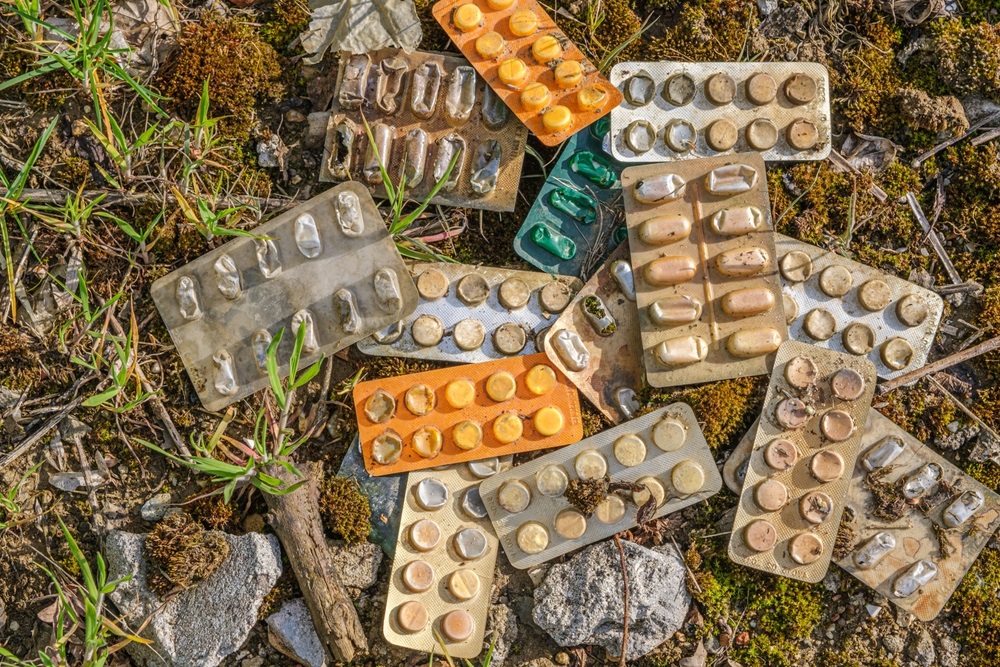
We may be familiar with the impact of plastic, agrochemical, and human waste, but what are the causes and consequences of pharmaceutical pollution?
It is nearly 62 years since Rachel Carson published Silent Spring1, disclosing the toxicity of pesticide use. A lifetime further on and we hear about pollution daily: the agrochemical pollution wiping out bees; the air pollution raising asthma rates; the sewage pollution in our rivers; and the tonnes of plastic polluting the oceans. We occasionally hear horror stories about oil spills or toxic chemical leaks yet rarely about the constant drip, drip, drip of pharmaceutical waste. When we think of chemicals, medicines aren’t the first thing that springs to mind, yet drugs and their metabolites are now widespread in the aquatic environment of groundwater, rivers, lakes, and the oceans.
Pharmaceuticals are biologically active chemicals. As they are designed to cause changes in living cells, they affect other living creatures, from fungi to fish, as well as our own bodies. Classed as ‘micropollutants’ they are also tiny. Too small to be filtered out in sewage systems, without special processes. In Europe, a lot of effluent grey water is released into large bodies of water (such as rivers) to dilute it, before being recaptured and recycled into drinking water. Climate change reduces the flow of water in rivers, and water scarcity prevents the proper dilution of micropollutants increasing the hazard level. With veterinary pharmaceutical waste much of it goes directly into rivers, bypassing even the most basic levels of remedial treatment.
To illustrate the scale of this, ten years ago, just under 9.5 million kilos of medically significant antibiotics were sold for use in food for animals compared to just under 3.5 million sold for human use – a ratio of nearly 3:1 animals:humans (2). Global meat production in 2027 is projected to be 15% higher than when those figures were recorded. Over the same period, global fish farming — another major source of pharmaceutical pollution — is expected to increase by 13.4%. In 2016, between 100 and 200 different types of pharmaceuticals were found in British surface water, ground water, tap and drinking water, leading Europe alongside Germany and Spain (3) and, globally, over 600 different products have been discovered in freshwater.
Pharmaceutical waste comes from three main areas: manufacturing, disposal and excretion.
Manufacturing
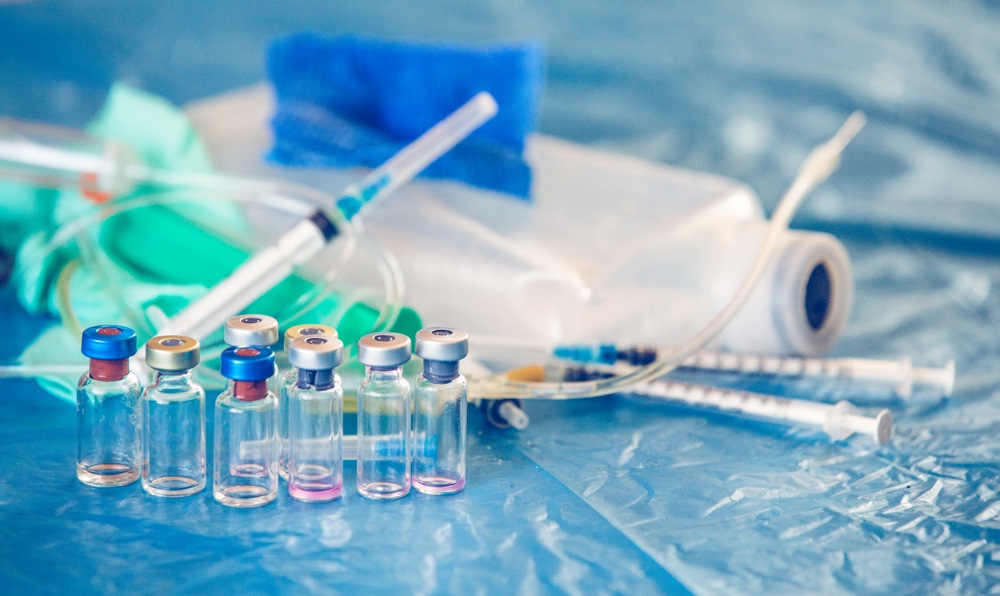
We like to think that factories are tightly controlled by legislation but standards vary considerably around the world. In 2007, for example, the wastewater of pharmaceutical factories in Patancheru (India), had higher concentrations of various medicines in the water than in the blood of patients on medication. Ciprofloxacin, a broad-spectrum antibiotic, was one million times greater than levels in treated urban wastewater and, at 44 kg of discharge a day, could have treated 44,000 people a day (4).
Closer to home, in 2016, University of Leeds scientists researched the presence of five drugs — ibuprofen, erythromycin, diclofenac, mefanamic acid and propanolol — in West Yorkshire rivers. The results were concerning. In the case of diclofenac, 46% of the samples contained more than double the limit set by the European Commission (5). In the last decade, Western countries have tightened the rules for manufacture surrounding biohazardous disposal, but this doesn’t apply to consumers.
Disposal
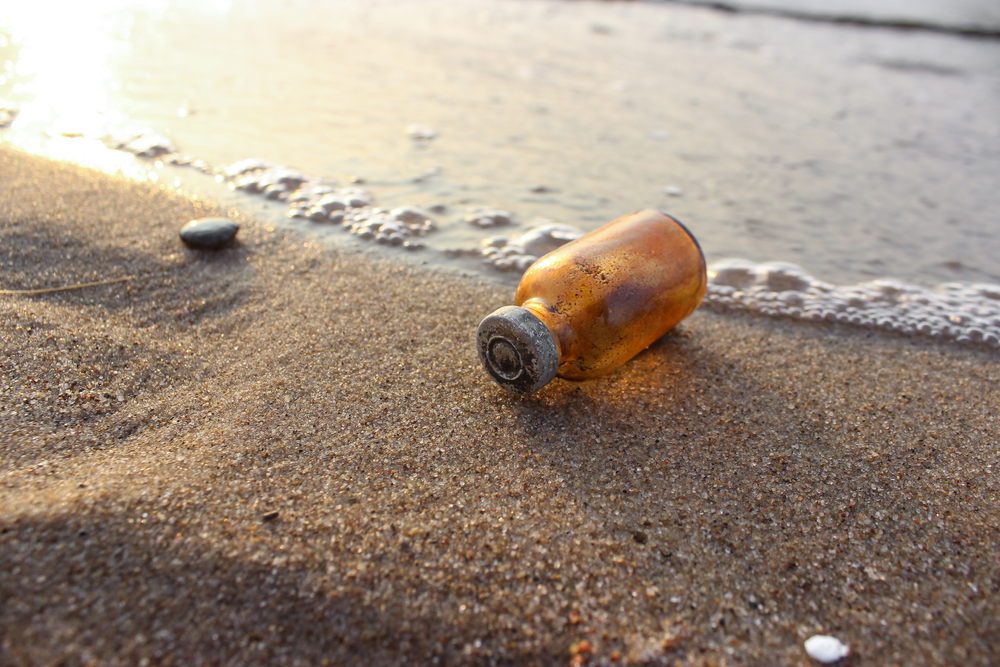
Pharmaceutical disposal waste includes drugs that are unused, often because the patient has got better or stopped taking them due to forgetfulness, side effects or doubts that they are working. Other reasons for drugs being unused include: unwanted repeat prescriptions; spoiled, damaged or contaminated; withdrawn or recalled; overstocked or past their expiry date. Around £300 million worth of medicine prescribed by the NHS go to waste every year — this includes both partially used and unused medicines — with a sixth of that coming from care homes. Statistics from the USA reckon that two out of three prescribed medicines remain unused. Where I live in Central Scotland, wasted medicines cost NHS Forth Valley approximately £1.2 million every year (6) (£20 million across NHS Scotland plus a cost of £500,000 a year to collect and destroy unused prescriptions). The advent of free prescriptions has also led to people stockpiling medicines that they don’t use.
The wrong disposal of unused medicines is a growing global problem. Most consumers put unused pills in the bin or down the toilet where they go direct to landfill sites or sewage plants. Even among educated populations over half are disposed of this way. Unwanted drugs should be returned to a chemist where they are collected by waste management companies, who then burn them at high temperatures to break down and destroy their active compounds, lowering the risk to wildlife and other organisms. What is left over — around 3% of the original mass — ends up in landfill. On one hand, incineration can significantly reduce the volume of waste going into landfills, in turn reducing their methane emissions. However, burning waste carries the potential of toxic gas emissions if not carried out in a modern incinerator with an advanced filtration system.
Hundreds of thousands of tonnes of hospital drug waste are generated in the UK each year, including used discarded drugs, intravenous lines, syringes, catheters and other drug-contaminated medical equipment. The NHS Clinical Waste Strategy (7) by NHS England has a waste segregation target set for 2026 but the deadline for appointment of dedicated hospital waste managers was only recent — 2023.
Excretion
Did you know that the bodies of humans and farm animals do not utilise all the active ingredients provided in a drug? What isn’t taken up leaves the body in urine or faeces that enters the wastewater system (if human), drains and rivers (if animal). It is estimated that the active ingredients are excreted unchanged through urine and faeces after taking them some 30% to 90% of the time8. DrugBank Online9 lists the excretion rates of some common drugs as follows:
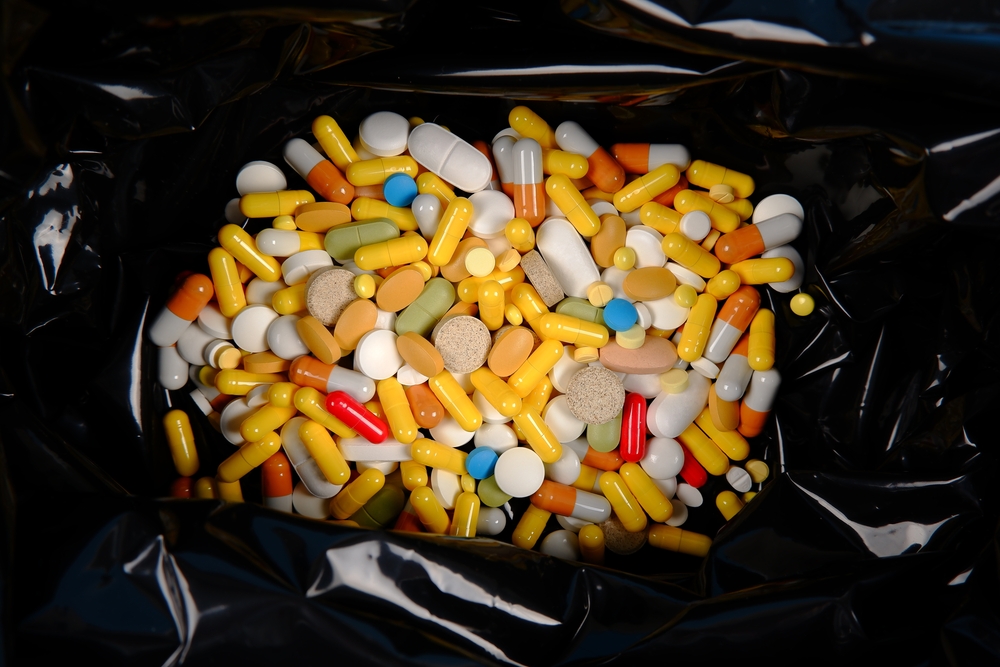
- Pain relief: ibuprofen 95%; paracetamol 85-95%; aspirin 10-85%
- Psychiatric medications: citalopram 23% urine, 10% faeces; fluoxetine <10%
- Antibiotics: amoxicillin 70-78%; cephalexin 90%; sulfamethoxazole 84%
- Antivirals: acyclovir 60%; valacyclovir 47%
- Heart medicines: atenolol 50%; clopidogrel 50% urine, 46% faeces; tadalafil 36% urine, 61% faeces
- Contraceptives: levonorgestrel 45% urine, 32% faeces
Take just one concerning example, that of the steroid hormones levonorgestrel found in most contraceptives. Globally, an estimated 151 million women of reproductive age use oral hormonal contraceptives (10) and steroid hormones are also found in devices like the Mirena coil. Researchers have known for decades that synthetic hormones — grouped under the heading xenoestrogens — in English rivers have caused the feminisation of aquatic species. This isn’t entirely due to the steroid oestrogens from drugs but also other oestrogen-like chemicals used in plastics, plasticisers, flame retardants, solvents, lubricants and pesticides. However, xenoestrogens reduce ovarian function in women and sperm count and sperm motility in men.
Levonorgestrel is a synthetic progestogen hormone found in the three contraceptive brands most commonly prescribed in the UK, Microgynon, Rigevidon and Ovranette; plus the mini-pills Norgeston and Cerazette; the Mirena coil and Plan B (the ‘morning-after pill’). Studies clearly prove it reduces fertility and sperm motility in male minnows, frogs, rats and, in a high enough quantity, human males. The amount in water is not a large dose for an adult male but the accumulated effects of this over a lifetime just isn’t known, particularly in unborn or infant males drinking water during the period of testicular development when semen quality is still taking place.
A meta-analysis of European studies collated in 2017 (11) found that sperm concentration had declined over the last 50 years with a mean average decrease of 33%. Some studies recorded a 50% decline and, in a Swiss study, 62% of men had suboptimal sperm. This could be more than just a coincidence.
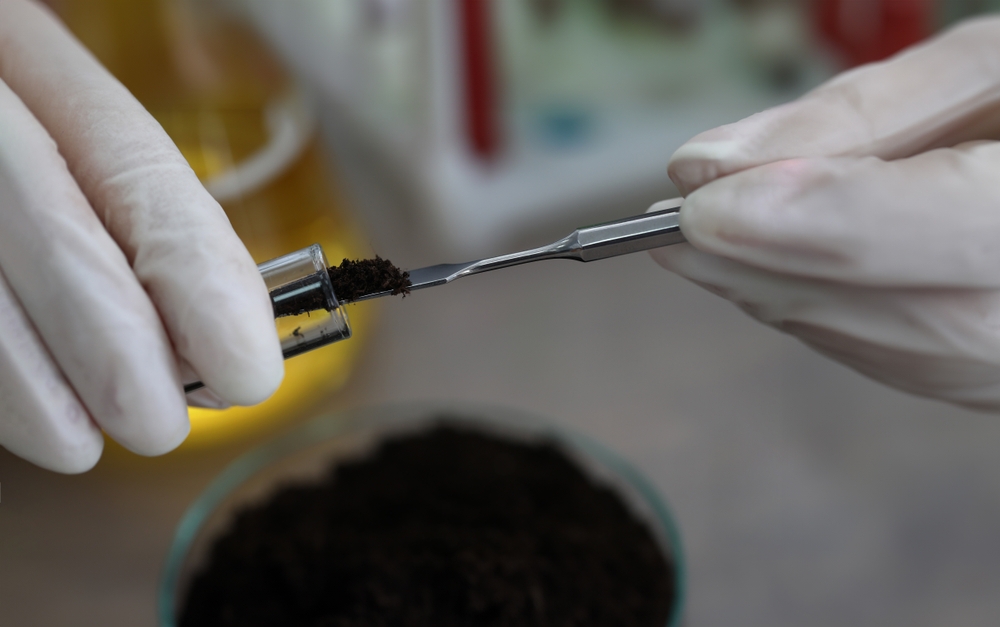
Chemotherapy drug excretion is another area of concern with cancer now affecting 1 in 2 people in the UK. These toxic drugs are often not included in waste water surveys, but those that do find tamoxifen and letrozole in high concentrations as they can only be significantly degraded with UV light irradiation (12). A human study on tamoxifen found nearly 27% of the dose excreted in urine and 25% in faeces (13). Chemotherapy drugs in body fluids can also carry risks for close family members.
No discussion would be complete without debating the concerns around antibiotic excretion. The root of the antibiotic word is –anti = against, –biotic = living organisms, therefore antibiotics will obviously destroy other life forms in the soil and water. Bacteria are fighting back and antibiotic resistance in medicine is one of the largest threats facing humanity today. In a large study, 757 samples were collected from sewage in 243 cities in 101 countries across seven key global regions. The average number of bacterial species found in each sample was 969, containing 557 different antimicrobial resistant genes (14).
By 2022, although the concentrations of most active pharmaceutical ingredients in the world’s rivers are still considered to be ‘too low to cause ecological effects’ nevertheless many, including sulfamethoxazole (antimicrobial), propranolol (β-blocker), loratadine (antihistamine), amitriptyline (antidepressant), citalopram (antidepressant), fexofenadine (antihistamine), verapamil (Ca channel blocker), and ketotifen (antihistamine) are consistently found at high levels that breach ecological guidelines (15).
Our global ecology is certainly affected: from the decline of vultures in Pakistan caused by diclofenac residues, cocaine found in salmon, to oxycodone and antidepressants found in mussels in Puget Sound on the American coast. Ultimately, as environmental regulations for emissions tend to be set on an individual basis, drug-by-drug, who really knows what the true environmental impact is of the complex cocktails now found in every drop of water?
References
- Carson, R. (1962). Silent Spring. Houghton Mifflin Company.
- OECD (2019), Pharmaceutical Residues in Freshwater: Hazards and Policy Responses, OECD Studies on Water, OECD Publishing, Paris, https://doi.org/10.1787/c936f42d-en
- aus der Beek, T., Weber, F. A., Bergmann, A., Hickmann, S., Ebert, I., Hein, A., & Küster, A. (2016). Pharmaceuticals in the environment–Global occurrences and perspectives. Environmental toxicology and chemistry, 35(4), 823–835. https://doi.org/10.1002/etc.3339
- Larsson D. G. (2014). Pollution from drug manufacturing: review and perspectives. Philosophical transactions of the Royal Society of London. Series B, Biological sciences, 369(1656), 20130571. https://doi.org/10.1098/rstb.2013.0571
- Kay, P., Hughes, S. R., Ault, J. R., Ashcroft, A. E., & Brown, L. E. (2017). Widespread, routine occurrence of pharmaceuticals in sewage effluent, combined sewer overflows and receiving waters. Environmental Pollution (1987), 220, 1447–1455. https://doi.org/10.1016/j.envpol.2016.10.087
- Forth Medical Group. (n.d.). Wasted medications. Retrieved April 2, 2024, from https://www.fmg.scot.nhs.uk/clinics-and-services/services/repeat-prescription-requests/wasted-medications/
- NHS England. (2023). NHS Clinical Waste Strategy (PR2159_i). https://www.england.nhs.uk/long-read/nhs-clinical-waste-strategy/
- González Peña, O. I., López Zavala, M. Á., & Cabral Ruelas, H. (2021). Pharmaceuticals Market, Consumption Trends and Disease Incidence Are Not Driving the Pharmaceutical Research on Water and Wastewater. International journal of environmental research and public health, 18(5), 2532. https://doi.org/10.3390/ijerph18052532
- DrugBank Online. (n.d.). DrugBank. Retrieved March 10, 2024, from https://go.drugbank.com/drugs
- Haakenstad, A., Angelino, O., Irvine, C. M., Bhutta, Z. A., Bienhoff, K., Bintz, C., … & Lozano, R. (2022). Measuring contraceptive method mix, prevalence, and demand satisfied by age and marital status in 204 countries and territories, 1970–2019: a systematic analysis for the Global Burden of Disease Study 2019. The Lancet, 400(10348), 295-327.
- Levine, H., Jørgensen, N., Martino-Andrade, A., Mendiola, J., Weksler-Derri, D., Mindlis, I., … & Swan, S. H. (2017). Temporal trends in sperm count: a systematic review and meta-regression analysis. Human reproduction update, 23(6), 646-659.
- Alitalo, O. S., Rantalainen, A. L., & Pellinen, J. (2022). Anticancer drugs gemcitabine, letrozole, and tamoxifen in municipal wastewater and their photodegradation in laboratory-scale UV experiments. Water, Air, & Soil Pollution, 233(8), 292.
- Kisanga, E. R., Mellgren, G., & Lien, E. A. (2005). Excretion of hydroxylated metabolites of tamoxifen in human bile and urine. Anticancer research, 25(6C), 4487-4492.
- Munk, P., Brinch, C., Møller, F. D., Petersen, T. N., Hendriksen, R. S., Seyfarth, A. M., et al. (2022). Genomic analysis of sewage from 101 countries reveals global landscape of antimicrobial resistance. Nature communications, 13(1), 7251. https://doi.org/10.1038/s41467-022-34312-7
- Wilkinson, J. L., Boxall, A. B. A., Kolpin, D. W., Leung, K. M. Y., Lai, R. W. S., Galbán-Malagón, C., et al. (2022). Pharmaceutical pollution of the world’s rivers. Proceedings of the National Academy of Sciences of the United States of America, 119(8), e2113947119. https://doi.org/10.1073/pnas.2113947119