Inflammation in the nervous system can lead to a plethora of problems. However, herbal medicines have a variety of actions that can help, this article explains how.
Introduction
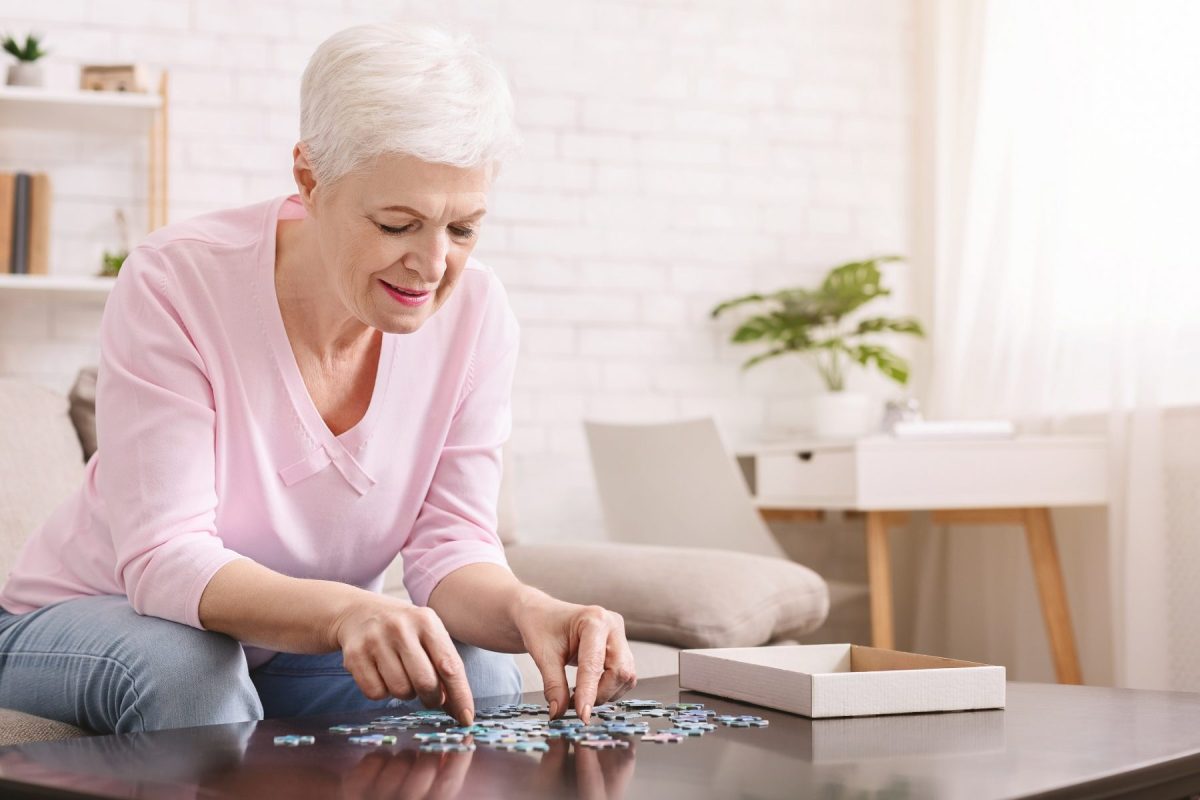
It was once thought that the brain and central nervous system (CNS) were largely protected by the ‘blood-brain barrier’ from immunological, inflammatory and infective processes in the wider body, and indeed from many medicines and plant constituents. This view now needs to be amended. Systemic inflammation or immune disease can disrupt this barrier, to provoke inflammatory processes within the CNS, with secondary complications there including protein aggregations, oxidative stress and disturbed mitochondrial function. This ‘neuroinflammation’ is now understood as the major factor in neurodegenerative diseases like Alzheimer’s, Parkinson’s, ALS and multiple sclerosis (1,2). It is also seen as contributing to mental diseases such as schizophrenia, clinical depression and bipolar disorder, (3) and increasingly to so far unexplained conditions like post-viral syndromes (including long Covid [4,5]) ME and other chronic fatigue syndromes (6) and fibromyalgia (7).
The prospect of finding remedies that could modulate such inflammatory processes is only just emerging from the laboratory, with all the attendant caveats, and there are few human studies yet published. However the weight of evidence for plant interventions has been building, (8,9,10) to such an extent now that there is a huge incentive for herbal practitioners and nutritional therapists to develop new approaches to the management of some of the most pressing problems of modern times (11).
Before we look at some of these prospects, it will be important to explore some of the mechanisms that are emerging as central to these conditions. They are changing much of what we learnt in school! There is a wide range of accessible scientific papers cited for further reading.
Fire in the brain
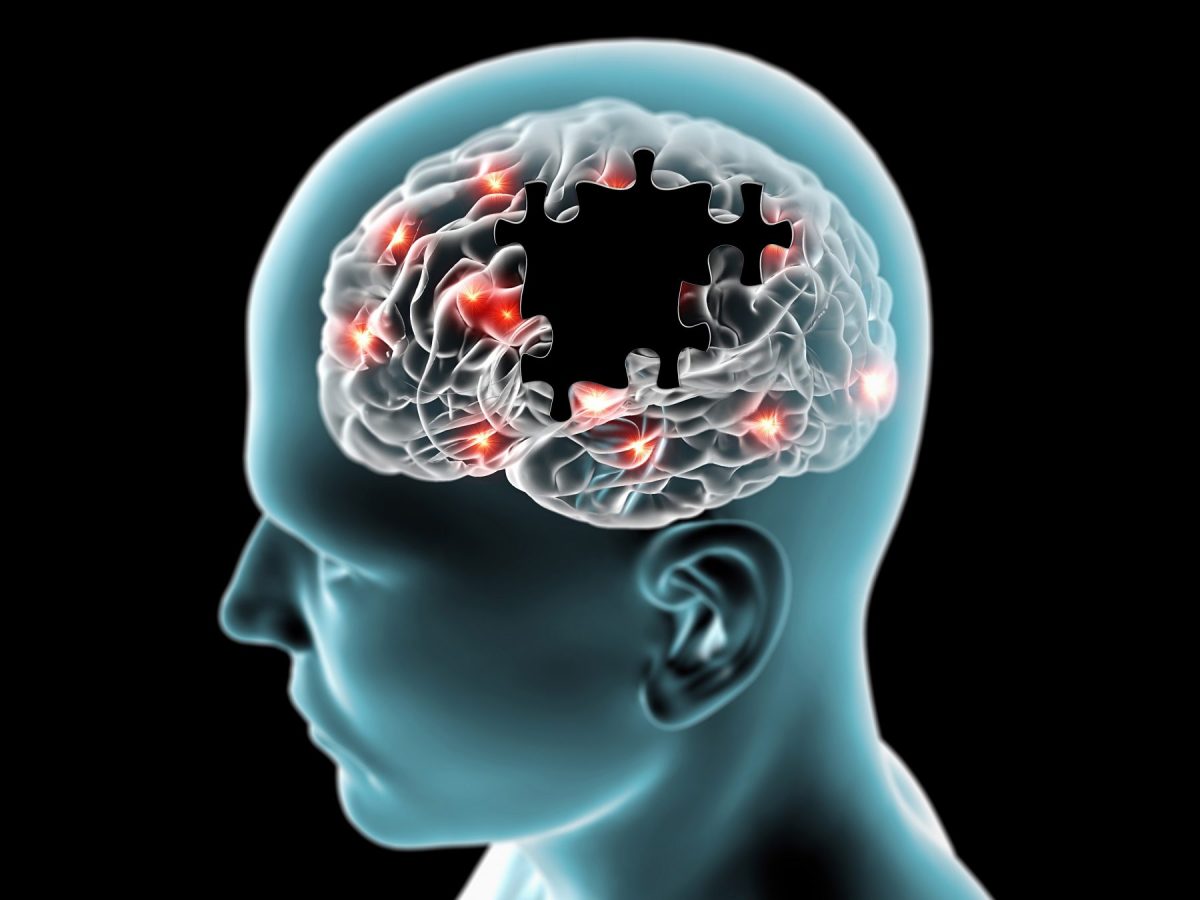
Neuroinflammation is the subject of increasing academic, medical and psychiatric attention (12). It is defined as an inflammatory response within the brain or spinal cord. Like other inflammation it is initially beneficial, removing harmful metabolites, inhibiting pathogens and promoting repair, but if persistent can lead to neurodegeneration(13). Key agents of this response include resident support cells (the ‘glia’: microglia and macroglia), endothelial cells at the blood-brain barrier, and immune cells migrating from the rest of the body. These cells can be ‘activated’ to generate a raft of inflammatory agents such as IL-1β (strongly associated with major depressive disorders as well as fatigue [14]), IL-6, IL-8, IL-33, TNF-α, CCL2, MMPs, substance P, reactive oxygen species, histamine and proteases, PAR-2, and NF-kB (15).
In a process referred to as ‘immunoexcitotoxicity’ these inflammatory agents can in turn excite glutamate receptors to increase reactive oxygen species/reactive nitrogen species, lipid peroxidation products, and prostaglandin activation, which then leads to dendritic retraction, synaptic injury, damage to microtubules, and mitochondrial suppression (16). Increasing evidence indicates that it is mitochondrial dysfunction, involving alterations in mitochondrial respiratory enzyme activities, oxidative stress, disrupted cell wall permeabilty, and enhanced apoptosis, that plays a particularly significant role in neuropathophysiological processes (17).
A key mechanism in preventing neuroinflammatory damage has emerged. There is increasing data on the pivotal role of one of the most powerful innate antioxidant mechanisms in the cell, Nrf2 (nuclear factor erythroid 2 related factor 2), against inflammatory processes in neurodegenerative disorders. The ‘Nrf2/ARE’ (Nrf2 bound to an antioxidant responsive element) signalling pathway has shown upregulation of antioxidant genes, inhibition of microglia-mediated inflammation, and improved mitochondrial function in neurodegenerative diseases (18). As will be seen below, polyphenols and other plant constituents are likely to protect against neurodegeneration through Nrf2 activation (19).
Microglia
Microglia are the main neuroinflammatory agents. They are the primary immune cells throughout the brain and CNS and comprise between 5-15% of the central nervous tissue population. Core functions include
- primary immune surveillance and other monitoring of the CNS environment;
- physiological housekeeping e.g migrating to injured sites, remodelling synapses, and maintaining myelin homeostasis; (20)
- macrophage-like protection against injurious stimuli such as pathogen-associated molecular patterns (PAMPs) and damage-associated molecular patterns (DAMPs), including with the production of inflammatory cytokines and chemokines.
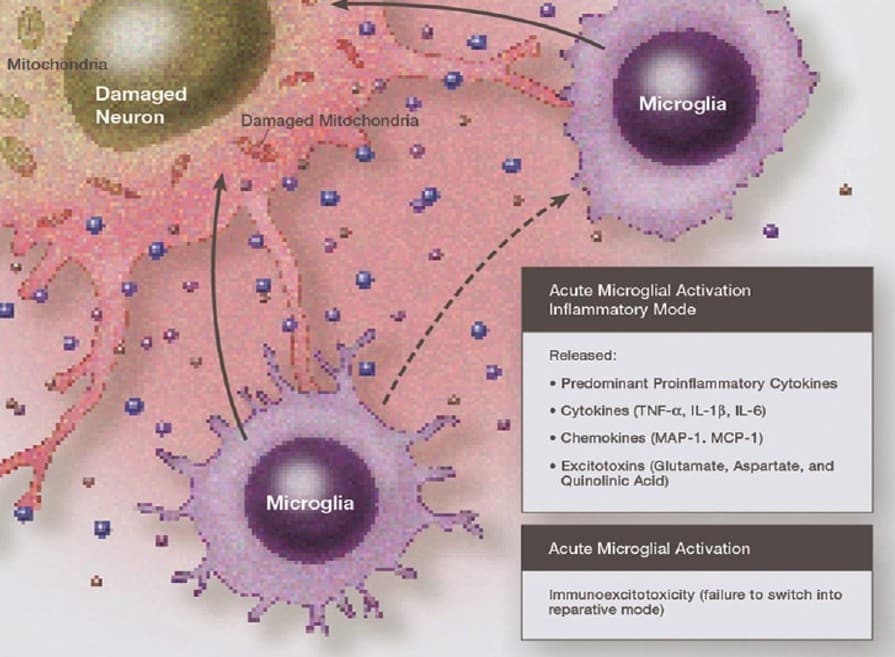
Microglia develop in the embryo as a variety of macrophage, but then migrate to the emerging nervous system (which they help to shape [21]) and thereafter their turnover appears to be wholly within the CNS. Turnover rates are low and this could account to susceptibility to the effects of age, (22) injury or stress (22).
Note that microglia are not to be confused with ‘macroglia’, notably astrocyctes, large star-shaped cells originating alongside neurons from neuroblastic tissue, and with complex supporting roles, including having profuse interactions with neuronal dendrites and synapses (perhaps millions per astrocyte). Astrocytes themselves however can become excited by activated microglia (via TNF-α-mediated increase in ATP secretion or activation with toll-like receptor (TLR) ligands [24]) and in turn can stimulate greater neurotransmitter activity. This observation is significant because pathological activation of microglia and alteration of neurotransmission are both early symptoms of most brain diseases.
Microglia vary considerably in their responses, with initial differentiation between ‘good’ and ‘bad’ types (26) now elaborated by the awareness that many different phenotypes and behaviours are present (27,28), complicating therapeutic interventions like anti-inflammatory drugs (29).
In health the relationship between neurons and microglia is mutually positive: healthy neurons produce calming agents, eg fractalkine, that prevent inappropriate microglial activation (30), while inactivated microglia generate neurotrophic agents such as brain-derived neurotrophic factor (BDNF) that are important for example in recovery from ischaemic stroke (31). The interactions are also dynamic and intimate. Microglial responses depend closely on neuronal activity (32) and include ‘pruning’ or ‘stripping’ of superfluous or dysfunctional synapses (33).
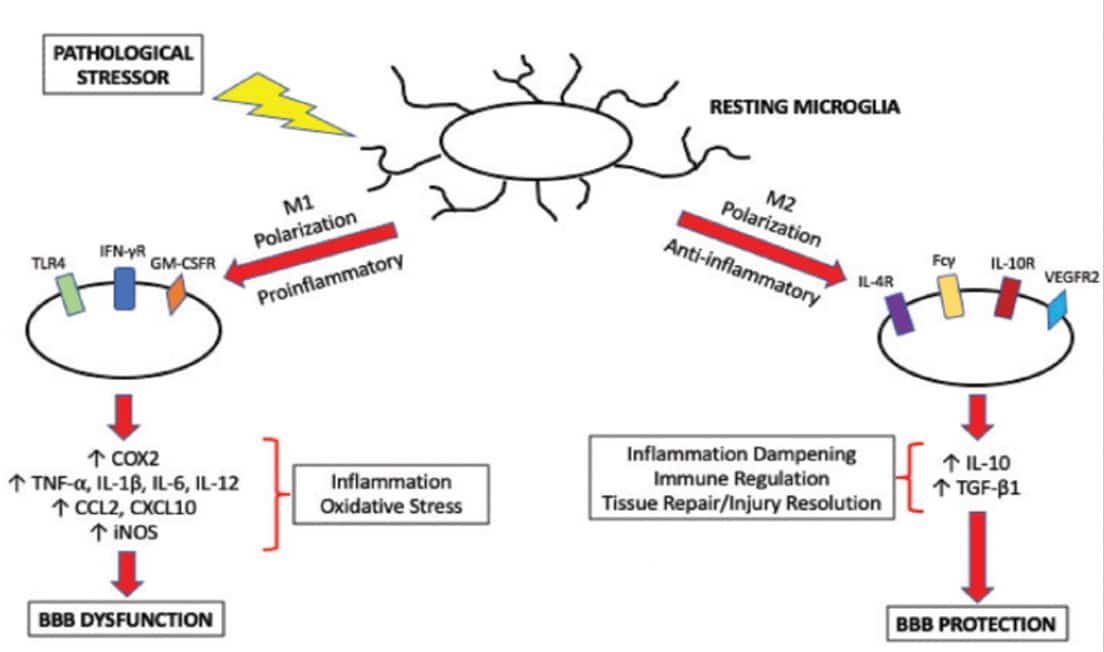
However following injury or disruption, especially with the inflammatory elements noted above, the resting spider-shaped microglia responsible for surveillance change to the amoeboid appearance associated with their activated state, and kick off production of interleukins and other cytokines, as well as a cascade of astrocytic activations.
Allowing for the complexities in microglial phenotypes referred to earlier there is no doubt that there is a polarisation of microglial activation
- to an M1 phenotype by exposure to inflammatory lipopolysaccharides (LPS) or IFN-γ, leading to the expression of pro-inflammatory cytokines notably IL-1β , or
- to an M2 phenotype following exposure to IL-4/IL-13, for resolution of inflammation and tissue repair mediated by TGF-β, IL-4 or IL-10. (34)
Factors that lead to M1-activation of microglia are likely to be central to neuroinflammatory disease. On the other hand therapeutic strategies that could favour M2 anti-inflammatory activation of microglia hold much therapeutic promise (35).
The blood brain barrier
The blood-brain barrier (BBB) is a highly specialized physical and biochemical barrier that separates the CNS from the peripheral circulation and is critical in maintaining its microenvironment. BBB disruption is implicated in a wide range of CNS pathologies including stroke, Alzheimer’s, multiple sclerosis, Parkinson’s and epilepsy.
Normal BBB protection is accomplished by specialized endothelial features:
- Tight junction protein complexes lock adjacent endothelial cell cytoskeletons with transmembrane proteins such as claudins, occludin, tricellulin and junctional adhesion molecules (JAMs).
- CNS endothelial cells express primary active transporters such as P-glycoprotein (P-gp), breast cancer resistance protein (BCRP), and multidrug resistance proteins (MRPs) that restrict the ability of many substances to access brain parenchyma (36).
- Disturbance in at least one of these transporters (P-gp) is often implicated in neuroinflammatory conditions (37). However, as this is expressed on the luminal surface of the cerebral blood vessels it provides an opportunity for blood-borne agents to affect BBB performance. St John’s wort has been suggested as having a benefit at this mechanism (38).
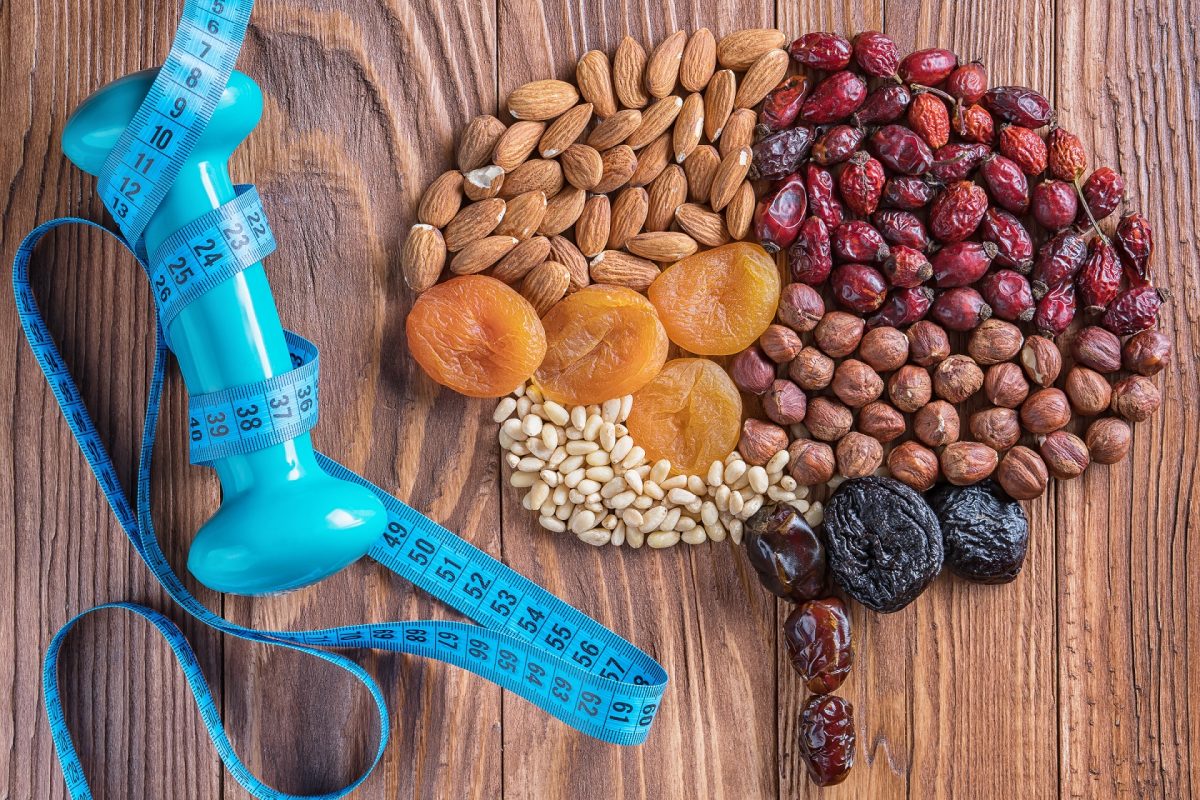
A key to understanding the BBB is to appreciate that it needs to be dynamic and adaptable to circumstances. There is a circulatory imperative: the brain requires constant high levels of glucose and oxygen as it has no stores itself. This requires that the brain microvasculature rapidly responds to environmental changes to match blood flow to brain metabolic demands, while still protecting the brain environment from threats. As well as core nutrients this barrier needs to allow for selective passage of immune cells as required (39). All this can only be done through coordinated cell activities, an insight that has given rise to the concept of a ‘neurovascular unit’ (40), a symbiosis of between endothelial cells, microvascular support cells (pericytes), glial cells, and neurons as well as extracellular components.
Of the glial cells the astrocytes, which cover the vast majority of blood vessel surfaces in the CNS, are known to have significant effect in supporting BBB integrity (41), in association with pericytes that also coat the blood vessels. It is the microglia again which appear to have particular influence on BBB responsiveness (43): many migrate to blood vessels walls as ‘perivascular microglia’ to monitor BBB integrity and the influx of blood solutes into brain parenchyma (44). The anti-inflammatory M2 activation of microglia is also associated with this protective activity (45). On the other hand microglia activated to the M1 phenotype by vascular exposure to pathophysiological stressors are likely to cause inflammatory damage to the BBB (46).
The implications for brain health in BBB breakdown are likely to be critical. Early consequences include cognitive dysfunction (47). Microvascular injury with BBB dysfunction and reduced cerebral blood flow has been cited as directly prompting the classic beta-amyloid protein accumulation in Alzheimer’s disease (48).
Role of the gut in neuroinflammation
There is increasing evidence that the gut microbiome and other aspects of gut health can play a critical part in the aetiology of neuroinflammatory conditions (49). Gut microbial dysbiosis leads to the secretion of amyloid and of lipopolysaccharides (LPS), which both disturb gastrointestinal permeability and the blood-brain barrier.

Amyloids (e.g. curli, tau, amyloid-beta, α-syn, and prion) derived from pro-inflammatory gut bacteria such as E.coli and species of Streptococcus, Staphylococcus, Salmonella, Mycobacteria, Klebsiella, Citrobacter, and Bacillus have been reported as initiating factors for the abnormal aggregation of amyloid-beta (Aβ) peptide in Alzheimer’s (50). Innate Aβ peptide production may actually be part of a healthy immune response against pathogenic microbes (51). However, bacterial amyloid mimics Aβ; through toll-like receptor TLR2 activation and pro-inflammatory mediator production (52) this can seed and elicit misfolding and aggregation of Aβ peptide, followed by microglial cell activation (53). Amyloidosis is confirmed as associated with higher gut levels of pro-inflammatory bacteria and lower anti-inflammatory bacteria (54).
Perhaps even more important for herbal therapeutics, M1 activation of microglia is also associated with the presence of bacterial lipopolysaccharide (LPS) absorbed from the gut (55,56). We shall see later that this is an obvious therapeutic target in managing neuroinflammation, and will also see more evidence of this in looking at depression.
Clearly there is a case in neuroinflammatory disorders for working on the gut environment. As one reviewer has noted: “consumption of prebiotics, probiotics, and synbiotics restored cognition … through gut-brain axis, leading to improved hippocampal plasticity, brain mitochondrial function, and decreased microglial activation” (57).
It is a reliable assumption in clinical practice that the most likely pressures in any chronic inflammatory condition in the body arise from the gut, and this is likely to apply too to all the problems of neuroinflammation that we have been describing. Finding ways to reduce the absorption of inflammatory agents and stealth pathogens, and to ameliorate immunological disturbances at the gut wall will always be a core role for the herbal practitioner. Supporting a healthy microbiome is emerging as the most important focus here and we explore the therapeutic prospects of working here in a separate piece in Herbal Reality.
Is depression caused by microglia?
Depression is increasingly viewed as an inflammatory disorder mediated by microglia (58), even being termed a ‘microgliopathy’ (59). It has been demonstrated that depressed patients present neuroinflammatory alterations, including changes in T-cell population (60), and have high blood levels of inflammatory cytokines (with levels of IL-1β, IL-10, and TNF-α correlated to depression severity, and IL-8 inversely so) (61). Levels of TSPO, a marker of microglial activation as measured by positron emission tomography (PET) scans, are elevated in the brains of those suffering major depressive episodes (62).
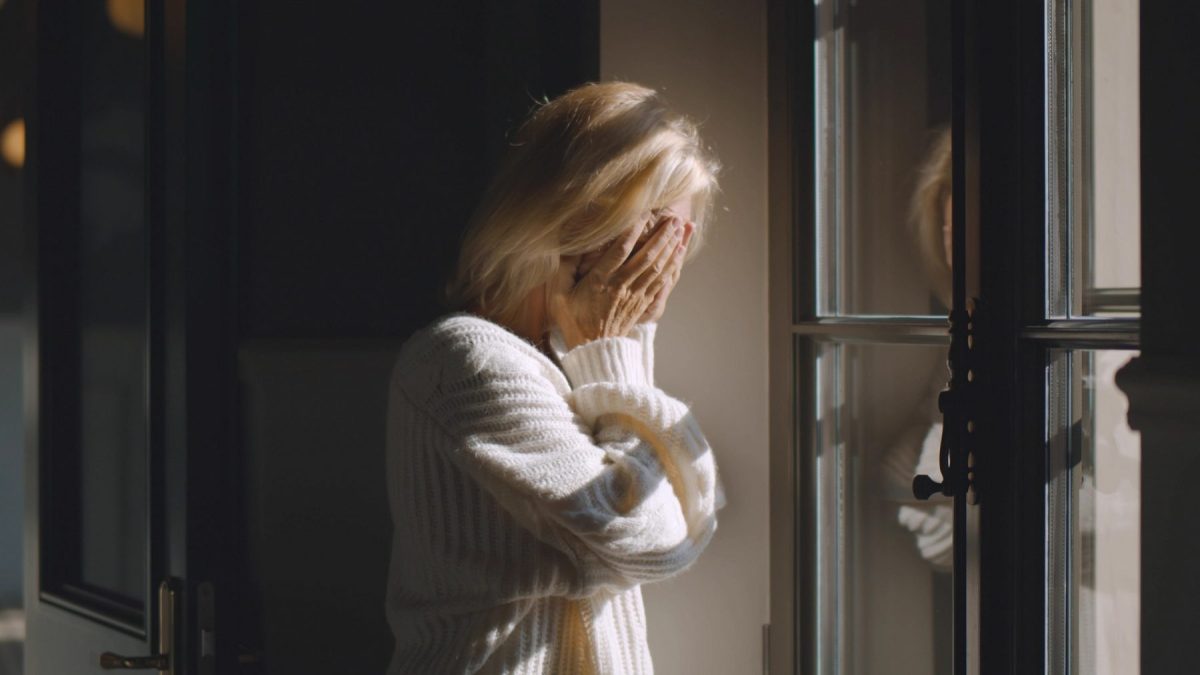
Depression is also strongly linked to infections, especially viral infections (63). These are likely related to microglial activation (64).
Interestingly for the herbal strategist, and restating the point above, provocations to depression can be linked also to a leaky gut wall: to high raised IgA/IgM responses to lipopolysaccharides (LPS) from Gram-negative gut bacteria (65,66). LPS are used in research to generate depressive behaviour, (67) and their depressant effects have been confirmed in human studies (68). Manipulations of the gut microbiota (and even vaccines) have been postulated as ways to prevent or treat depression (69).
It is however interesting to note that vulnerability to inflammatory factors appears to be modified by pre-existing socio-behavioural factors. A placebo-controlled study in 115 healthy adults of the effects of an infusion of gut derived LPS (in this case an endotoxin from E. coli), showed that expected increases in proinflammatory cytokines and subsequent depressed mood were moderated by baseline levels of perceived stress, sensitivity to social disconnection, and the severity of symptoms of anxiety and depression. These background factors were associated with increased activation of pro-inflammatory transcription control pathways (e.g. NF-κB) in response to endotoxin (70). In other words, inflammatory causes of depression do not act in isolation from other prevailing factors.
Neuroinflammation in chronic fatigue
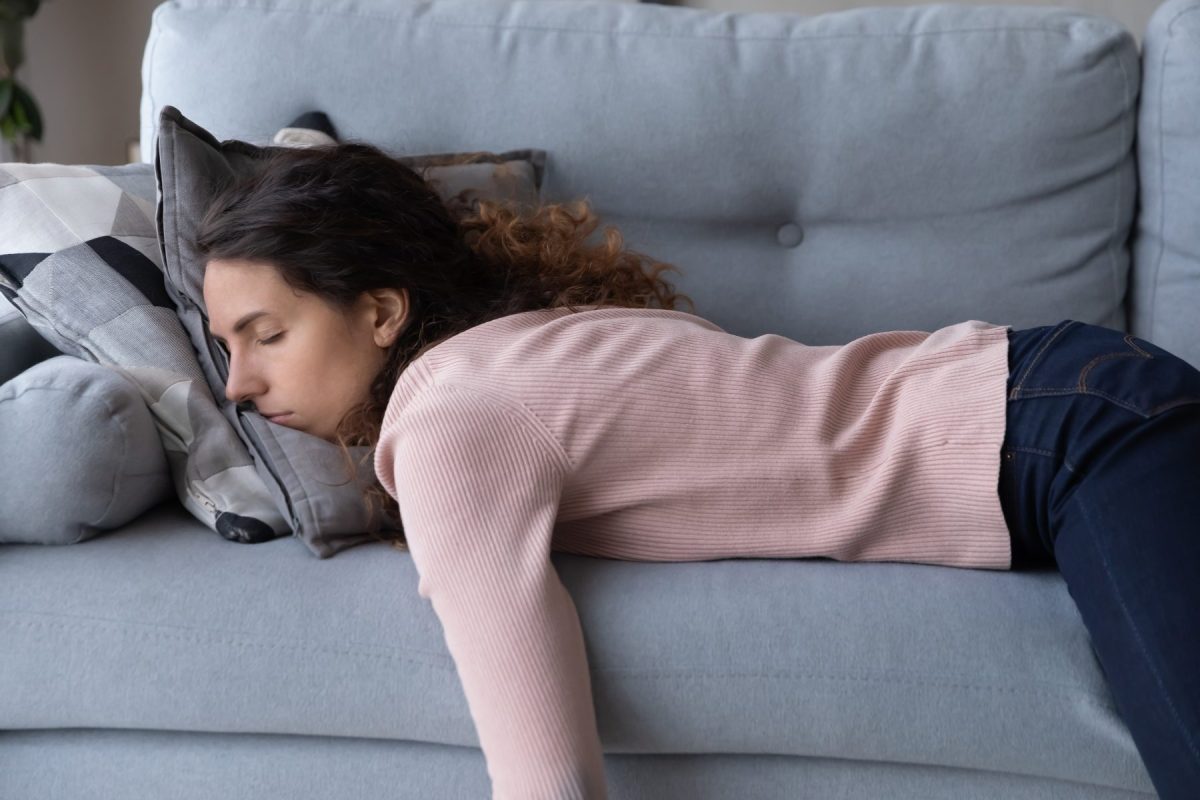
The obvious fatigue that follows infections as well as inflammatory and immunological diseases has been linked to increased levels of pro-inflammatory cytokines. These include IL-1, IL-6, TNF-α and IFNα; activation of the TLR Cycle by pathogen-associated and damage-associated molecular patterns (‘PAMPs’ and ‘DAMPs’), altered glutaminergic and dopaminergic neurotransmission, mitochondrial dysfunctions, and oxidative stress-induced defects in the sodium-potassium pump (71). In various laboratory models viral infection has been demonstrated as able to increase blood brain barrier permeability, microglia/macrophage activation through Toll-like receptor 3 (TLR3) signalling, and upregulation of the serotonin transporter in astrocytes so reducing extracellular serotonin (5-HT) levels (72).
There have been further suggestions that chronic fatigue syndromes can have autoimmune origins, with increased immune activity against 5-HT, associated with activation of immuno-inflammatory pathways and bacterial translocation (73).
Methodological limitations so far hamper firm conclusion’s but activation of microglia and astrocytes is increasingly considered a leading factor in the case of chronic fatigue syndrome (74).
Plant-based approaches to reducing neuroinflammation
It is becoming clear that unhealthy modern lifestyles are contributing to neuroinflammatory disorders (75). The obverse of that is that there are many positive dietary measures that could be adopted, (76,77) and in particular the prospects for the use of spices, (78) herbs and dietary plants in modulating neuroinflammation are increasingly promising (79,80,81,82,83,84).
There is the real prospect that plants may turn out to be better agents at reducing neuroinflammatory damage than new anti-inflammatory medicines. They have the huge advantage that most can be safely consumed over many months and years, forming part of the diet as well as in a herbal regime. Below are some of the more interesting subjects of current research, many published just before this article was written and certainly to be seen in increasing volume in the future.
Polyphenols
Polyphenols exert numerous biological effects likely to help protect the ‘neurovascular unit’, including anti-aggregatory platelet activity, the vasodilatory effects of nitric oxide generation, free radical scavenging properties, and acting on the signalling cascades implicated in endothelial apoptosis. Such benefits have been identified for protection against stroke damage (85), and even in reducing later protein aggregate formation in Alzheimer’s and Parkinson’s diseases (86,87). Various protective functions have been identified for flavonoids (88,89,90,91,92). In the case of cocoa polyphenols these have also been associated with their role in modulating intestinal immunity (93). Other promising remedies with high polyphenol content include turmeric, rosemary, green tea, red grapes and ginkgo, outlined below.
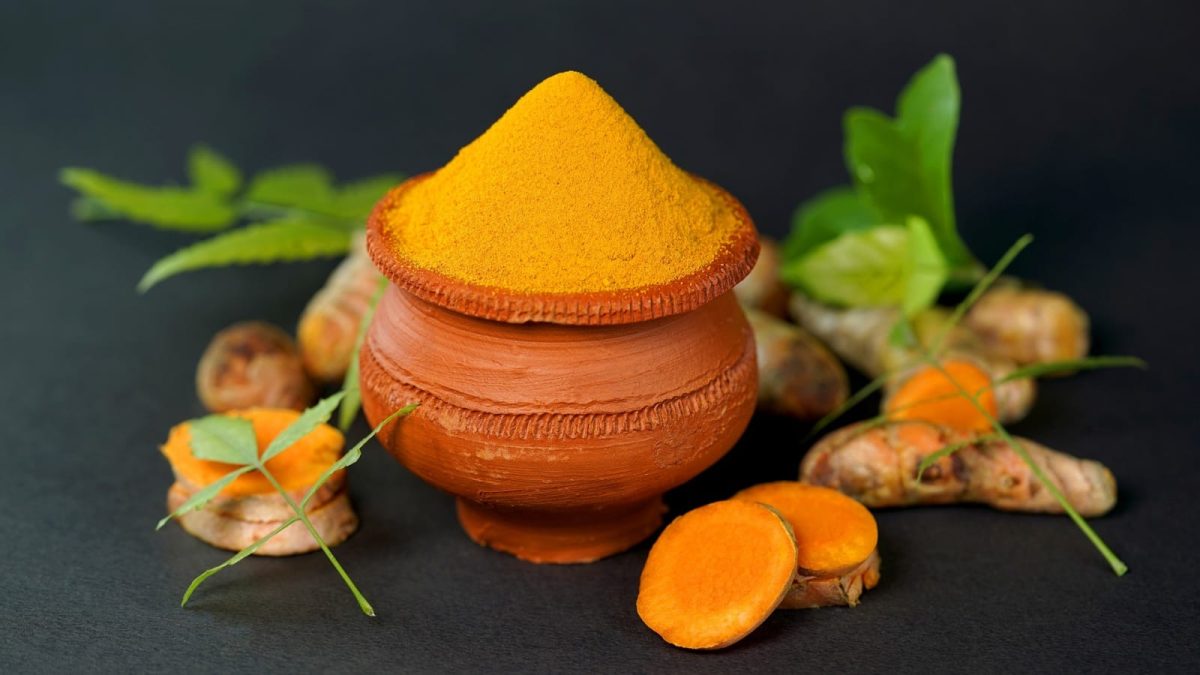
Turmeric (Curcuma longa)
Turmeric’s prominent polyphenol complex curcumin has been shown to reduce levels of TLR4 and its downstream effectors including NF-κB, IRF3, MyD88, and TIRF that are associated with stimulated astrocytes (94), and to have a wide range of potential neuroprotective properties (95). However the very poor absorption of curcumin across the blood-brain barrier on top of its core absorption problems across the gut wall means that much laboratory research on the role of naturally-presented curcumin in nerve tissue should be discounted in clinical practice (96). There are some promising leads for a readily absorbed turmeric constituent ar-turmerone (97). However a more promising mechanism is the capacity of turmeric and curcumin to reduce the absorption of inflammatory bacterial LPS from the gut (98,99,100). As has already been noted in this article, bacterial LPS is a potent factor in neuroinflammation and this may turn out to be the most exciting mechanism for benefits of turmeric in alleviating neuroinflammation.
Rosemary (Rosmarinus officinalis)
Rosemary leaves contain two diterpenes, carnosic acid and carnosol, which account for most of its observed antioxidant, anti-inflammatory, and anti-carcinogenic activities in the laboratory. They also demonstrate neuroprotective effects and reduction in neuroinflammatory activity in both in vitro and in vivo, in some cases more intensely than resveratrol or sulforaphane (see below) (101). Rosemary also includes caffeic acid and its ester rosmarinic acid as important bioactive constituents. Rosmarinic acid demonstrates neuroprotective effects in different models of neuroinflammation and neurodegeneration, as well as chemical-induced neurotoxicity and oxidative stress (102).
Green tea (Camellia sinensis) and EGCG
As well as high polyphenol content the most prominent constituents in green tea research are the catechins, especially epigallocatechin-3-gallate (EGCG). These have been shown to have a wide range of neuroprotective benefits across many models including in clinical studies, and justifies the value of probably both green and black teas as the most widely consumed fluids around the world (103,104,105,106,107,108,109).
Ginkgo (Ginkgo biloba)
A standardised ginkgo leaf extract (EGb761) was shown to reverse microglial activation and release of inflammatory agents caused by interference to CNS circulation (110), to significantly inhibit astrocyte activation after ischaemic stroke (111), to have stabilising effects on LPS-activated microglia in vitro (112), and to reduce other neuroinflammatory activity (113). One of ginkgo’s terpene lactones, ginkgolide B, has demonstrated neuroprotective effects in several models of neurological diseases (114), including in reducing the deposition of protein plaques (115). Although these are laboratory studies using specific derivatives of ginkgo leaf they point to promising effects in managing the microvascular elements of neurological disease in humans.
Red grapes, red wine and resveratrol
Resveratrol, a stilbene polyphenol found in many plant species, and notably red grape skins and seeds, is actually capable of crossing the blood-brain barrier and has been found, at least in part (116), to have multiple biological properties, including attenuating mitochondrial dysfunction (117), preventing neurological disorders, reducing neuroinflammatory processes, inhibiting apoptosis (118,119), reducing microglial activation (120), and stabilising astrocyte activity (121). Most interestingly, in relation to the specific neuroinflammatory processes associated with liver damage (hepatic encephalopathy), the neurological benefits of resveratrol could be mediated by changes to the gut microbiota (122).
Broccoli and sulforaphane
Sulforaphane is an active constituent in various species of brassica and is provided in broccoli seed sprouts supplements. It is a prominent activator of Nrf2 (nuclear factor erythroid 2 related factor 2 – outlined above). In the CNS sulforaphane has shown protective potential against a wide range of neuropathies via promotion of neurogenesis or inhibition of oxidative stress and neuroinflammation (123,124).
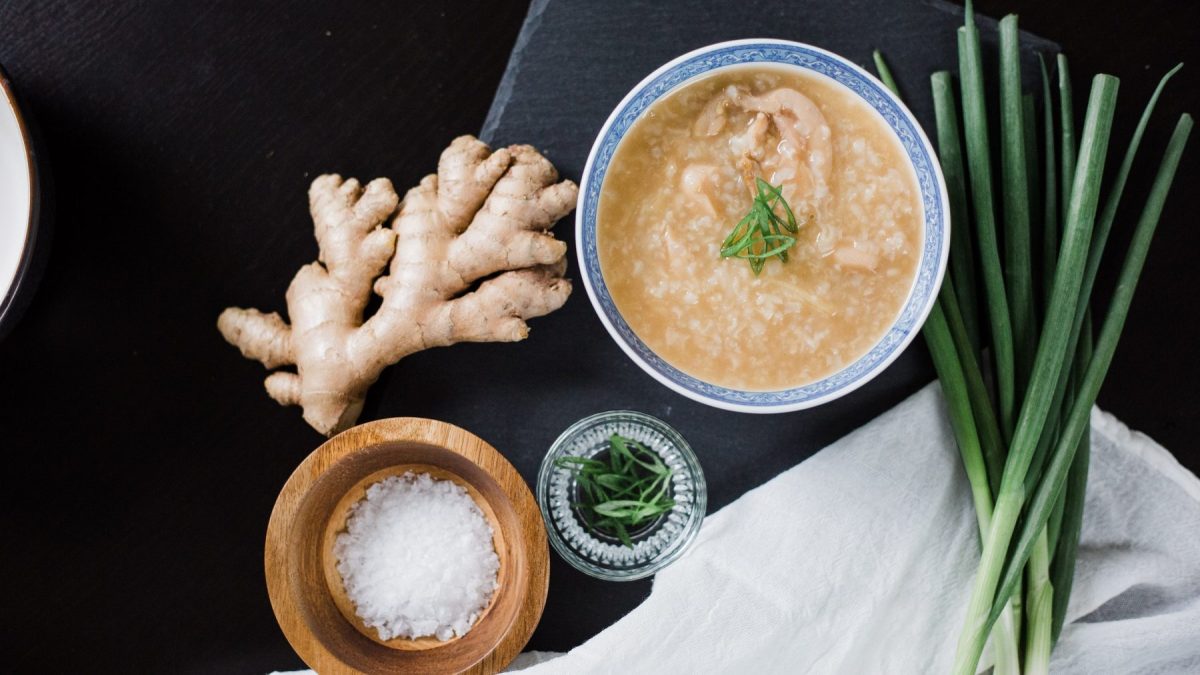
Ginger (Zingiber officinalis)
This common food ingredient looks another promising component of an anti-neuroinflammatory regime (125,126,127). As with turmeric there are prospects that some of these effects may be mediated via changes in the gut microbiota (128). There are studies that implicate both shogaols (129,130) and gingerols (131) in these benefits.
Cinnamon (Cinnamomum spp)
Extract of cinnamon and cinnaldehyde inhibit abnormal protein plaque formations in a number of models, have effects against multiple oxidative stress and pro-inflammatory pathways, modulate endothelial functions and attenuate vascular cell adhesion molecules (132). Cinnamic acid has been shown to help shift microglial activation to the M2 phenotype (133).
There are also pointers to neuroprotective effects for other herbs (134):
- Ashwagandha (Withania somnifera) has been shown to have a range of effects reducing LPS-induced neuroinflammation (135), and ameliorated neurotoxic complications of high fat diets (137).
- Brahmi (Bacopa monnieri) inhibits the release of inflammatory cytokines from microglial cells and inhibits enzymes associated with inflammation in the brain (137), as well as other activities consistent with protection against neurodegenerative diseases (128).
- Saffron (Crocus sativa) is showing evidence of inhibiting amyloid-beta aggregation, preventing plaque and neurofibrillary tangle formation, and in other ways reducing neuroinflammation (139).
Wu wei zi – Chinese magnolia berry (Schisandra chinensis)
Prominent constituents of this important TCM remedy are dibenzocyclooctadiene lignans such as schisandrins: various studies have reported that the crude extracts and the isolated pure lignan components effectively protect the neuronal cell damage (140).
References
- Guo S, Wang H, Yin Y. (2022) Microglia Polarization From M1 to M2 in Neurodegenerative Diseases. Front Aging Neurosci. 14: 815347. doi: 10.3389/fnagi.2022.815347.
- Kempuraj D, Thangavel R, Natteru PA, et al. (2016) Neuroinflammation Induces Neurodegeneration. J Neurol Neurosurg Spine. 1(1):1003. PMID: 28127589
- Menculini G, Chipi E, Paolini Paoletti F, et al. (2021) Insights into the Pathophysiology of Psychiatric Symptoms in Central Nervous System Disorders: Implications for Early and Differential Diagnosis. Int J Mol Sci. 22(9): 4440. doi: 10.3390/ijms22094440
- Toniolo S, Scarioni M, Di Lorenzo F, et al; Management Group of the EAN Dementia and Cognitive Disorders Scientific Panel. (2021) Dementia and COVID-19, a Bidirectional Liaison: Risk Factors, Biomarkers, and Optimal Health Care. J Alzheimers Dis. 82(3): 883-898. doi: 10.3233/JAD-210335.
- Song WJ, Hui CKM, Hull JH, et al. (2021) Confronting COVID-19-associated cough and the post-COVID syndrome: role of viral neurotropism, neuroinflammation, and neuroimmune responses. Lancet Respir Med. 9(5): 533-544. doi: 10.1016/S2213-2600(21)00125-9.
- Nakatomi Y, Mizuno K, Ishii A, et al. (2014) Neuroinflammation in Patients with Chronic Fatigue Syndrome/Myalgic Encephalomyelitis: An ¹¹C-(R)-PK11195 PET Study. J Nucl Med. 55(6): 945-50. doi: 10.2967/jnumed.113.131045.
- Arnett SV, Clark IA. (2012) Inflammatory fatigue and sickness behaviour – lessons for the diagnosis and management of chronic fatigue syndrome. J Affect Disord. 141(2-3): 130-42. doi: 10.1016/j.jad.2012.04.004.
- Seo EJ, Fischer N, Efferth T. (2018) Phytochemicals as inhibitors of NF-κB for treatment of Alzheimer’s disease. Pharmacol Res. 129: 262-273. doi: 10.1016/j.phrs.2017.11.030.
- Cicero AFG, Fogacci F, Banach M. (2018) Botanicals and phytochemicals active on cognitive decline: The clinical evidence. Pharmacol Res. 130: 204-212. doi: 10.1016/j.phrs.2017.12.029.
- Hügel HM. (2015) Brain Food for Alzheimer-Free Ageing: Focus on Herbal Medicines. Adv Exp Med Biol. 863: 95-116. doi: 10.1007/978-3-319-18365-7_5.
- DiSabato DJ, Quan N, Godbout JP. (2016) Neuroinflammation: the devil is in the details. J Neurochem. 139, 2:136-153. doi: 10.1111/jnc.13607
- Bullmore E (2018) The Inflamed Mind: A Radical New Approach to Depression. Short Books, London.
- Wyss-Coray T, Mucke L. (2002) Inflammation in neurodegenerative disease–a double-edged sword. Neuron. 35 (3): 419-32. doi: 10.1016/s0896-6273(02)00794-8
- Roerink, M.E., van der Schaaf, M.E., Dinarello, C.A. et al. 2017) Interleukin-1 as a mediator of fatigue in disease: a narrative review. J Neuroinflammation 14,16. doi.org/10.1186/s12974-017-0796-7
- Subhramanyam CS, Wang C, Hu Q, Dheen ST. (2019) Microglia-mediated neuroinflammation in neurodegenerative diseases. Semin Cell Dev Biol. 94: 112-120. doi: 10.1016/j.semcdb.2019.05.004
- Blaylock RL, Maroon J. (2011) Immunoexcitotoxicity as a central mechanism in chronic traumatic encephalopathy-A unifying hypothesis. Surg Neurol Int. 2: 107. doi: 10.4103/2152-7806.83391.
- Kumar A, Singh A. (2015) A review on mitochondrial restorative mechanism of antioxidants in Alzheimer’s disease and other neurological conditions. Front Pharmacol. 6: 206. doi: 10.3389/fphar.2015.00206.
- Saha S, Buttari B, Profumo E, et al. (2022) A Perspective on Nrf2 Signaling Pathway for Neuroinflammation: A Potential Therapeutic Target in Alzheimer’s and Parkinson’s Diseases. Front Cell Neurosci. 15: 787258. doi: 10.3389/fncel.2021.787258
- Naoi M, Wu Y, Shamoto-Nagai M, Maruyama W. (2019) Mitochondria in Neuroprotection by Phytochemicals: Bioactive Polyphenols Modulate Mitochondrial Apoptosis System, Function and Structure. Int J Mol Sci. 20(10): 2451. doi: 10.3390/ijms20102451
- Kabba JA, Xu Y, Christian H, et al. (2018) Microglia: Housekeeper of the Central Nervous System. Cell Mol Neurobiol. 38(1): 53-71. doi: 10.1007/s10571-017-0504-2.
- Sierra A, Encinas JM, Deudero JJ, et al.(2010) Microglia shape adult hippocampal neurogenesis through apoptosis-coupled phagocytosis. Cell Stem Cell. 7(4): 483-95. doi: 10.1016/j.stem.2010.08.014
- Mosher KI, Wyss-Coray T. (2014) Microglial dysfunction in brain aging and Alzheimer’s disease. Biochem Pharmacol. 88(4): 594-604. doi: 10.1016/j.bcp.2014.01.008
- Ajami B, Bennett JL, Krieger C, et al. (2007) Local self-renewal can sustain CNS microglia maintenance and function throughout adult life. Nat Neurosci. 10 (12): 1538-43. doi: 10.1038/nn2014
- Ma D, Jin S, Li E, et al. (2013) The neurotoxic effect of astrocytes activated with toll-like receptor ligands. J Neuroimmunol. 254(1-2): 10-8. doi: 10.1016/j.jneuroim.2012.08.010.
- Pascual O, Ben Achour S, Rostaing P, et al. (2012) Microglia activation triggers astrocyte-mediated modulation of excitatory neurotransmission. Proc Natl Acad Sci U S A. 109(4): E197-205. doi: 10.1073/pnas.1111098109.
- Luo XG, Chen SD. (2012) The changing phenotype of microglia from homeostasis to disease. Transl Neurodegener. 1(1): 9. doi: 10.1186/2047-9158-1-9.
- Wolf SA, Boddeke HW, Kettenmann H. (2017) Microglia in Physiology and Disease. Annu Rev Physiol. 79: 619-643. doi: 10.1146/annurev-physiol-022516-034406
- Kwon HS, Koh SH. (2020). Neuroinflammation in neurodegenerative disorders: the roles of microglia and astrocytes. Transl Neurodegener. 9 (1), 42. https://doi.org/10.1186/s40035-020-00221-2
- Bachiller S, Jiménez-Ferrer I, Paulus A, et al. (2018) Microglia in Neurological Diseases: A Road Map to Brain-Disease Dependent-Inflammatory Response. Front Cell Neurosci.12: 488. doi: 10.3389/fncel.2018.00488
- Sheridan GK, Murphy KJ. (2013) Neuron-glia crosstalk in health and disease: fractalkine and CX3CR1 take centre stage. Open Biol. 3(12): 130181. doi: 10.1098/rsob.130181.
- García-Culebras A, Durán-Laforet V, Peña-Martínez C, et al. (2018) Myeloid cells as therapeutic targets in neuroinflammation after stroke: Specific roles of neutrophils and neutrophil-platelet interactions. J Cereb Blood Flow Metab. 38(12): 2150-2164. doi: 10.1177/0271678X18795789
- Tremblay MÈ, Lowery RL, Majewska AK. (2010) Microglial interactions with synapses are modulated by visual experience. PLoS Biol. 8(11): e1000527. doi: 10.1371/journal.pbio.1000527.
- Kettenmann H, Kirchhoff F, Verkhratsky A. (2013) Microglia: new roles for the synaptic stripper. Neuron 77(1): 10-8. doi: 10.1016/j.neuron.2012.12.023.
- Orihuela R, McPherson CA, Harry GJ. (2016) Microglial M1/M2 polarization and metabolic states. Br J Pharmacol. 173(4): 649-65. doi: 10.1111/bph.13139
- Subramaniam SR and Federoff HJ. (2017) Targeting microglial activation states as a therapeutic avenue in Parkinson’s disease. Front Aging Neurosci 9: 176.
- Liebner S, Dijkhuizen RM, Reiss Y, et al. (2018) Functional morphology of the blood-brain barrier in health and disease. Acta Neuropathol. 135(3): 311-336. doi: 10.1007/s00401-018-1815-1
- Cirrito JR, Deane R, Fagan AM, et al. (2005) P-glycoprotein deficiency at the blood-brain barrier increases amyloid-beta deposition in an Alzheimer disease mouse model. J Clin Invest. 115(11): 3285-90. doi: 10.1172/JCI25247.
- Pahnke J, Fröhlich C, Paarmann K, et al. (2014) Cerebral ABC transporter-common mechanisms may modulate neurodegenerative diseases and depression in elderly subjects. Arch Med Res. 45(8): 738-43. doi: 10.1016/j.arcmed.2014.10.010
- Engelhardt B, Ransohoff RM. (2012) Capture, crawl, cross: the T cell code to breach the blood-brain barriers. Trends Immunol. 33(12): 579-89. doi: 10.1016/j.it.2012.07.004.
- Iadecola C. (2017) The neurovascular unit coming of age: a journey through neurovascular coupling in health and disease. Neuron 96: 17–42
- Willis CL, Nolan CC, Reith SN, et al. (2004) Focal astrocyte loss is followed by microvascular damage, with subsequent repair of the blood-brain barrier in the apparent absence of direct astrocytic contact. Glia 45: 325–337.
- Bell RD, Winkler EA, Sagare AP, et al. (2010) Pericytes control key neurovascular functions and neuronal phenotype in the adult brain and during brain aging. Neuron 68: 409–427.
- Ronaldson PT, Davis TP. (2020) Regulation of blood-brain barrier integrity by microglia in health and disease: A therapeutic opportunity. J Cereb Blood Flow Metab. 40 (1_suppl): S6-S24. doi: 10.1177/0271678X20951995.
- Halder SK and Milner R. (2019) A critical role for microglia in maintaining vascular integrity in the hypoxic spinal cord. Proc Natl Acad Sci U S A 116: 26029–26037
- Zhang L, Lu X, Gong L, Cui L, et al. (2021) Tetramethylpyrazine Protects Blood-Spinal Cord Barrier Integrity by Modulating Microglia Polarization Through Activation of STAT3/SOCS3 and Inhibition of NF-кB Signaling Pathways in Experimental Autoimmune Encephalomyelitis Mice. Cell Mol Neurobiol. 41(4):717-731. doi: 10.1007/s10571-020-00878-3
- Haruwaka K, Ikegami A, Tachibana Y, et al. (2019) Dual microglia effects on blood brain barrier permeability induced by systemic inflammation. Nat Commun. 10: 5816.
- Nation DA, Sweeney MD, Montagne A, et al. (2019) Blood-brain barrier breakdown is an early biomarker of human cognitive dysfunction. Nat Med 25: 270–276
- Sweeney MD, Sagare AP and Zlokovic BV. (2018) Blood-brain barrier breakdown in Alzheimer disease and other neurodegenerative disorders. Nat Rev Neurol 14: 133–150.
- Goyal D, Ali SA, Singh RK. (2021) Emerging role of gut microbiota in modulation of neuroinflammation and neurodegeneration with emphasis on Alzheimer’s disease. Prog Neuropsychopharmacol Biol Psychiatry.106: 110112. doi: 10.1016/j.pnpbp.2020.110112
- Friedland RP, Chapman MR. (2017) The role of microbial amyloid in neurodegeneration. PLoS Pathog. 13(12):e1006654. doi: 10.1371/journal.ppat.1006654.
- Kumar DK, Choi SH, Washicosky KJ, et al. (2016) Amyloid-β peptide protects against microbial infection in mouse and worm models of Alzheimer’s disease. Sci Transl Med. 8(340): 340ra72. doi: 10.1126/scitranslmed.aaf1059
- Cerovic M, Forloni G, Balducci C. (2019) Neuroinflammation and the Gut Microbiota: Possible Alternative Therapeutic Targets to Counteract Alzheimer’s Disease? Front Aging Neurosci. 11: 284. doi: 10.3389/fnagi.2019.00284.
- Kesika P, Suganthy N, Sivamaruthi BS, Chaiyasut C. (2021) Role of gut-brain axis, gut microbial composition, and probiotic intervention in Alzheimer’s disease. Life Sci. 264: 118627. doi: 10.1016/j.lfs.2020.118627.
- Cattaneo A, Cattane N, Galluzzi S, et al INDIA-FBP Group. (2017) Association of brain amyloidosis with pro-inflammatory gut bacterial taxa and peripheral inflammation markers in cognitively impaired elderly. Neurobiol Aging. 49: 60-68. doi: 10.1016/j.neurobiolaging.2016.08.019.
- Sumi N, Nishioku T, Takata F, et al. (2010) Lipopolysaccharide-activated microglia induce dysfunction of the blood-brain barrier in rat microvascular endothelial cells co-cultured with microglia. Cell Mol Neurobiol 30: 247–253
- Maes M, Twisk FN, Kubera M, et al. (2012) Increased IgA responses to the LPS of commensal bacteria is associated with inflammation and activation of cell-mediated immunity in chronic fatigue syndrome. J Affect Disord. 136(3): 909-17. doi: 10.1016/j.jad.2011.09.010
- Chunchai T, Thunapong W, Yasom S, et al. Decreased microglial activation through gut-brain axis by prebiotics, probiotics, or synbiotics effectively restored cognitive function in obese-insulin resistant rats. J Neuroinflammation. 15(1): 11. doi: 10.1186/s12974-018-1055-2.
- Yirmiya R, Rimmerman N, Reshef R. (2015) Depression as a microglial disease. Trends Neurosci. 38(10): 637-658. doi: 10.1016/j.tins.2015.08.001.
- Yirmiya R, Rimmerman N, Reshef R. (2015) Depression as a microglial disease. Trends Neurosci. 38(10): 637-658. doi: 10.1016/j.tins.2015.08.001.
- Maes M, Stevens W, DeClerck L et al. (1992) Immune disorders in depression: higher T helper/T suppressor-cytotoxic cell ratio. Acta Psychiatr Scand. 86(6): 423-31. doi: 10.1111/j.1600-0447.1992.tb03292.x.
- Zou W, Feng R, Yang Y (2018) Changes in the serum levels of inflammatory cytokines in antidepressant drug-naïve patients with major depression. PLoS ONE 13(6): e0197267. doi:10.1371/journal.pone.0197267
- Holmes SE, Hinz R, Conen S, et al. (2018) Elevated Translocator Protein in Anterior Cingulate in Major Depression and a Role for Inflammation in Suicidal Thinking: A Positron Emission Tomography Study. Biol Psychiatry. 83(1): 61-69. doi: 10.1016/j.biopsych.2017.08.005.
- Coughlin, S.S. (2012) Anxiety and Depression: Linkages with Viral Diseases. Public Health Rev 34,7. doi:10.1007/BF03391675
- Chaves-Filho AJM, Macedo DS, de Lucena DF, Maes M. (2019) Shared microglial mechanisms underpinning depression and chronic fatigue syndrome and their comorbidities. Behav Brain Res. 372: 111975. doi: 10.1016/j.bbr.2019.111975
- Maes M, Kubera M, Leunis JC. (2008) The gut-brain barrier in major depression: intestinal mucosal dysfunction with an increased translocation of LPS from gram negative enterobacteria (leaky gut) plays a role in the inflammatory pathophysiology of depression. Neuro Endocrinol Lett. 29 (1): 117-24. PMID: 18283240.
- Stevens BR, Goel R, Seungbum K, et al (2018) Increased human intestinal barrier permeability plasma biomarkers zonulin and FABP2 correlated with plasma LPS and altered gut microbiome in anxiety or depression. Gut67:1555-1557. doi: 10.1136/gutjnl-2017-314759
- Remus, JL, Dantzer, R (2016) Inflammation Models of Depression in Rodents: Relevance to Psychotropic Drug Discovery, Int J Neuropsychopharmacology, 19, 9, pyw028, doi: 10.1093/ijnp/pyw028
- Sandiego CM, Gallezot JD, Pittman B, et al. (2015) Imaging robust microglial activation after lipopolysaccharide administration in humans with PET. Proc Natl Acad Sci U S A. 112(40): 12468-73. doi: 10.1073/pnas.1511003112
- Rook GA, Raison CL, Lowry CA. (2012) Can we vaccinate against depression? Drug Discov Today. 17(9-10): 451-8. doi: 10.1016/j.drudis.2012.03.018.
- Irwin MR, Cole S, Olmstead R, et al. (2019) Moderators for depressed mood and systemic and transcriptional inflammatory responses: a randomized controlled trial of endotoxin. Neuropsychopharmacology. 44(3): 635-641. doi: 10.1038/s41386-018-0259-6.
- Morris, G., Berk, M., Galecki, P. et al. (2016) The Neuro-Immune Pathophysiology of Central and Peripheral Fatigue in Systemic Immune-Inflammatory and Neuro-Immune Diseases. Mol Neurobiol 53, 1195–1219. doi: 10.1007/s12035-015-9090-9
- Noda M, Ifuku M, Hossain MS, Katafuchi T. (2018) Glial Activation and Expression of the Serotonin Transporter in Chronic Fatigue Syndrome. Front Psychiatry. 9: 589. doi: 10.3389/fpsyt.2018.00589.
- Maes M, Ringel K, Kubera M, et al. (2013) In myalgic encephalomyelitis/chronic fatigue syndrome, increased autoimmune activity against 5-HT is associated with immuno-inflammatory pathways and bacterial translocation. J Affect Disord. 150(2):223-30. doi: 10.1016/j.jad.2013.03.029.
- VanElzakker MB, Brumfield SA, Lara Mejia PS. (2019) Neuroinflammation and Cytokines in Myalgic Encephalomyelitis/Chronic Fatigue Syndrome (ME/CFS): A Critical Review of Research Methods. Front Neurol. 9: 1033. doi: 10.3389/fneur.2018.01033.
- Popa-Wagner A, Dumitrascu DI, Capitanescu B, et al. (2020) Dietary habits, lifestyle factors and neurodegenerative diseases. Neural Regen Res. 15(3): 394-400. doi: 10.4103/1673-5374.266045.
- Szczechowiak K, Diniz BS, Leszek J. (2019) Diet and Alzheimer’s dementia – Nutritional approach to modulate inflammation. Pharmacol Biochem Behav. 184: 172743. doi: 10.1016/j.pbb.2019.172743.
- Hornedo-Ortega R, Cerezo AB, de Pablos RM, et al. (2018) Phenolic Compounds Characteristic of the Mediterranean Diet in Mitigating Microglia-Mediated Neuroinflammation. Front Cell Neurosci. 12: 373. doi: 10.3389/fncel.2018.00373
- Mirmosayyeb O, Tanhaei A, Sohrabi HR, et al. (2017) Possible Role of Common Spices as a Preventive and Therapeutic Agent for Alzheimer’s Disease. Int J Prev Med. 8: 5. doi: 10.4103/2008-7802.199640.
- Zhu T, Wang L, Wang LP, Wan Q. (2022) Therapeutic targets of neuroprotection and neurorestoration in ischemic stroke: Applications for natural compounds from medicinal herbs. Biomed Pharmacother. 148: 112719. doi: 10.1016/j.biopha.2022.112719.
- Fumia A, Cicero N, Gitto M, et al. (2021) Role of nutraceuticals on neurodegenerative diseases: neuroprotective and immunomodulant activity. Nat Prod Res.: 1-18. doi: 10.1080/14786419.2021.2020265
- Patil P, Thakur A, Sharma A, Flora SJS. (2020) Natural products and their derivatives as multifunctional ligands against Alzheimer’s disease. Drug Dev Res. 81(2): c165-183. doi: 10.1002/ddr.21587.
- Wang J, Song Y, Chen Z, Leng SX. (2018) Connection between Systemic Inflammation and Neuroinflammation Underlies Neuroprotective Mechanism of Several Phytochemicals in Neurodegenerative Diseases. Oxid Med Cell Longev:1972714. doi: 10.1155/2018/1972714.
- Seo EJ, Fischer N, Efferth T. (2018) Phytochemicals as inhibitors of NF-κB for treatment of Alzheimer’s disease. Pharmacol Res. 129: 262-273. doi: 10.1016/j.phrs.2017.11.030.
- Morgan LA, Grundmann O. (2017) Preclinical and Potential Applications of Common Western Herbal Supplements as Complementary Treatment in Parkinson’s Disease. J Diet Suppl. 14(4): 453-466. doi: 10.1080/19390211.2016.1263710.
- Curin Y, Ritz MF, Andriantsitohaina R. (2006) Cellular mechanisms of the protective effect of polyphenols on the neurovascular unit in strokes. Cardiovasc Hematol Agents Med Chem. 4(4): 277-88. doi: 10.2174/187152506778520691.
- Revi N, Rengan AK. (2021) Impact of dietary polyphenols on neuroinflammation-associated disorders. Neurol Sci. 42(8): 3101-3119. doi: 10.1007/s10072-021-05303-1.
- Freyssin A, Page G, Fauconneau B, Rioux Bilan A. (2018) Natural polyphenols effects on protein aggregates in Alzheimer’s and Parkinson’s prion-like diseases. Neural Regen Res. 13(6): 955-961. doi: 10.4103/1673-5374.233432.
- Hamsalakshmi, Alex AM, Arehally Marappa M, et al. (2022) Therapeutic benefits of flavonoids against neuroinflammation: a systematic review. Inflammopharmacology. doi: 10.1007/s10787-021-00895-8.
- Spencer JP. (2007). The interactions of flavonoids within neuronal signalling pathways. Genes Nutr, 2(3), 257–273. doi: 10.1007/s12263-007-0056-z
- Tili E, Michaille JJ. (2016) Promiscuous Effects of Some Phenolic Natural Products on Inflammation at Least in Part Arise from Their Ability to Modulate the Expression of Global Regulators, Namely microRNAs. Molecules. 21(9): 1263. doi: 10.3390/molecules21091263.
- Rahimifard M, Maqbool F, Moeini-Nodeh S, et al. (2017) Targeting the TLR4 signaling pathway by polyphenols: A novel therapeutic strategy for neuroinflammation. Ageing Res Rev. 36: 11-19. doi: 10.1016/j.arr.2017.02.004.
- Subedi L, Gaire BP. (2021) Phytochemicals as regulators of microglia/macrophages activation in cerebral ischemia. Pharmacol Res. 165: 105419. doi: 10.1016/j.phrs.2021.105419.
- Magrone T, Russo MA, Jirillo E. (2017) Cocoa and Dark Chocolate Polyphenols: From Biology to Clinical Applications. Front Immunol. 8: 677. doi: 10.3389/fimmu.2017.00677.
- Yu S, Wang X, He X, et al. (2016) Curcumin exerts anti-inflammatory and antioxidative properties in 1-methyl-4-phenylpyridinium ion (MPP(+))-stimulated mesencephalic astrocytes by interference with TLR4 and downstream signaling pathway. Cell Stress Chaperones. 21(4): 697-705. doi: 10.1007/s12192-016-0695-3.
- Cianciulli A, Calvello R, Ruggiero M, Panaro MA. (2022) Inflammaging and Brain: Curcumin and Its Beneficial Potential as Regulator of Microglia Activation. Molecules. 27(2): 341. doi: 10.3390/molecules27020341
- Yavarpour-Bali H, Ghasemi-Kasman M, Pirzadeh M. (2019) Curcumin-loaded nanoparticles: a novel therapeutic strategy in treatment of central nervous system disorders. Int J Nanomedicine. 14: 4449-4460. doi: 10.2147/IJN.S208332.
- Hucklenbroich J, Klein R, Neumaier B, et al. (2014) Aromatic-turmerone induces neural stem cell proliferation in vitro and in vivo. Stem Cell Res Ther. 5(4): 100. doi: 10.1186/scrt500.
- Ghosh SS, He H, Wang J, et al. (2018) Curcumin-mediated regulation of intestinal barrier function: The mechanism underlying its beneficial effects. Tissue Barriers. 6(1): e1425085. doi: 10.1080/21688370.2018.1425085.
- Ganji A, Farahani I, Saeedifar AM, et al. (2021) Protective Effects of Curcumin against Lipopolysaccharide-Induced Toxicity. Curr Med Chem. 28(33): 6915-6930. doi: 10.2174/0929867328666210525124707.
- Shen L, Ji HF. (2019) Bidirectional interactions between dietary curcumin and gut microbiota. Crit Rev Food Sci Nutr. 59(18): 2896-2902. doi: 10.1080/10408398.2018.1478388.
- de Oliveira MR. (2016) The Dietary Components Carnosic Acid and Carnosol as Neuroprotective Agents: a Mechanistic View. Mol Neurobiol. 53(9): 6155-6168. doi: 10.1007/s12035-015-9519-1.
- Nabavi SF, Tenore GC, Daglia M, et al. (2015) The cellular protective effects of rosmarinic acid: from bench to bedside. Curr Neurovasc Res. 12(1): 98-105. doi: 10.2174/1567202612666150109113638
- Ide K, Matsuoka N, Yamada H, et al. (2018) Effects of Tea Catechins on Alzheimer’s Disease: Recent Updates and Perspectives. Molecules. 23(9): 2357. doi: 10.3390/molecules23092357.
- Renaud J, Nabavi SF, Daglia M, et al. (2015) Epigallocatechin-3-Gallate, a Promising Molecule for Parkinson’s Disease? Rejuvenation Res. 18(3): 257-69. doi: 10.1089/rej.2014.1639
- Özduran G, Becer E, Vatansever HS. (2021) The Role and Mechanisms of Action of Catechins in Neurodegenerative Diseases. J Am Coll Nutr. 1-8. doi: 10.1080/07315724.2021.1981487.
- Fernandes L, Cardim-Pires TR, Foguel D, Palhano FL. (2021) Green Tea Polyphenol Epigallocatechin-Gallate in Amyloid Aggregation and Neurodegenerative Diseases. Front Neurosci. 15: 718188. doi: 10.3389/fnins.2021.718188.
- Gonçalves PB, Sodero ACR, Cordeiro Y. (2021) Green Tea Epigallocatechin-3-gallate (EGCG) Targeting Protein Misfolding in Drug Discovery for Neurodegenerative Diseases. Biomolecules. 11(5): 767. doi: 10.3390/biom11050767.
- Lee YJ, Choi DY, Yun YP, et al. (2013) Epigallocatechin-3-gallate prevents systemic inflammation-induced memory deficiency and amyloidogenesis via its anti-neuroinflammatory properties. J Nutr Biochem. 24(1): 298-310. doi: 10.1016/j.jnutbio.2012.06.011
- Li J, Ye L, Wang X, et al. (2012) (-)-Epigallocatechin gallate inhibits endotoxin-induced expression of inflammatory cytokines in human cerebral microvascular endothelial cells. J Neuroinflammation. 9: 161. doi: 10.1186/1742-2094-9-161.
- Kim MS, Bang JH, Lee J, et al. Ginkgo biloba L. extract protects against chronic cerebral hypoperfusion by modulating neuroinflammation and the cholinergic system. Phytomedicine. 23(12): 1356-1364. doi: 10.1016/j.phymed.2016.07.013.
- Zhang Y, Liu J, Yang B, et al. Ginkgo biloba Extract Inhibits Astrocytic Lipocalin-2 Expression and Alleviates Neuroinflammatory Injury via the JAK2/STAT3 Pathway After Ischemic Brain Stroke. Front Pharmacol. 9: 518. doi: 10.3389/fphar.2018.00518.
- Gargouri B, Carstensen J, Bhatia HS, et al. (2018) Anti-neuroinflammatory effects of Ginkgo biloba extract EGb761 in LPS-activated primary microglial cells. Phytomedicine.44: 45-55. doi: 10.1016/j.phymed.2018.04.009.
- Wan W, Zhang C, Danielsen M, et al. (2016) EGb761 improves cognitive function and regulates inflammatory responses in the APP/PS1 mouse. Exp Gerontol. 81: 92-100. doi: 10.1016/j.exger.2016.05.007.
- Yin JJ, He Y, An J, et al. (2020) Dynamic Balance of Microglia and Astrocytes Involved in the Remyelinating Effect of Ginkgolide B. Front Cell Neurosci. 13: 572. doi: 10.3389/fncel.2019.00572
- Bate C, Salmona M, Williams A. (2004) Ginkgolide B inhibits the neurotoxicity of prions or amyloid-beta1-42. J Neuroinflammation. 1(1): 4. doi: 10.1186/1742-2094-1-4.
- Yang AJT, Bagit A, MacPherson REK. (2021) Resveratrol, Metabolic Dysregulation, and Alzheimer’s Disease: Considerations for Neurogenerative Disease. Int J Mol Sci. 22(9): 4628. doi: 10.3390/ijms22094628.
- Jardim FR, de Rossi FT, Nascimento MX, et al. (2018) Resveratrol and Brain Mitochondria: a Review. Mol Neurobiol. 55(3): 2085-2101. doi: 10.1007/s12035-017-0448-z.
- Miguel CA, Noya-Riobó MV, Mazzone GL, et al. (2021) Antioxidant, anti-inflammatory and neuroprotective actions of resveratrol after experimental nervous system insults. Special focus on the molecular mechanisms involved. Neurochem Int. 150: 105188. doi: 10.1016/j.neuint.2021.105188.
- Dos Santos MG, Schimith LE, André-Miral C, et al. (2022) Neuroprotective Effects of Resveratrol in In vivo and In vitro Experimental Models of Parkinson’s Disease: a Systematic Review. Neurotox Res 40(1): 319-345 doi: 10.1007/s12640-021-00450-x
- Huang J, Huang N, Xu S, et al. (2021) Signaling mechanisms underlying inhibition of neuroinflammation by resveratrol in neurodegenerative diseases. J Nutr Biochem. 88: 108552. doi: 10.1016/j.jnutbio.2020.108552.
- Fan R, Zhang Y, Botchway BOA, Liu X. (2021) Resveratrol Can Attenuate Astrocyte Activation to Treat Spinal Cord Injury by Inhibiting Inflammatory Responses. Mol Neurobiol. 58(11): 5799-5813. doi: 10.1007/s12035-021-02509-4.
- Kim YK, Song J. (2021) Therapeutic Applications of Resveratrol in Hepatic Encephalopathy through Its Regulation of the Microbiota, Brain Edema, and Inflammation. J Clin Med. 10(17): 3819. doi: 10.3390/jcm10173819.
- Uddin MS, Mamun AA, Jakaria M, et al. (2020) Emerging promise of sulforaphane-mediated Nrf2 signaling cascade against neurological disorders. Sci Total Environ. 707: 135624. doi: 10.1016/j.scitotenv.2019.135624
- Huang C, Wu J, Chen D, et al. (2019) Effects of sulforaphane in the central nervous system. Eur J Pharmacol. 853: 153-168. doi: 10.1016/j.ejphar.2019.03.010
- Schepici G, Contestabile V, Valeri A, Mazzon E. (2021) Ginger, a Possible Candidate for the Treatment of Dementias? Molecules. 26(18): 5700. doi: 10.3390/molecules26185700.
- Talebi M, İlgün S, Ebrahimi V, et al. (2021) Zingiber officinale ameliorates Alzheimer’s disease and Cognitive Impairments: Lessons from preclinical studies. Biomed Pharmacother. 133: 111088. doi: 10.1016/j.biopha.2020.111088.
- Choi JG, Kim SY, Jeong M, Oh MS. (2018) Pharmacotherapeutic potential of ginger and its compounds in age-related neurological disorders. Pharmacol Ther. 182: 56-69. doi: 10.1016/j.pharmthera.2017.08.010.
- Wang X, Zhang D, Jiang H, et al. (2021) Gut Microbiota Variation With Short-Term Intake of Ginger Juice on Human Health. Front Microbiol. 11: 576061. doi: 10.3389/fmicb.2020.576061.
- Moon M, Kim HG, Choi JG, et al. (2014) 6-Shogaol, an active constituent of ginger, attenuates neuroinflammation and cognitive deficits in animal models of dementia. Biochem Biophys Res Commun. 449(1): 8-13. doi: 10.1016/j.bbrc.2014.04.121.
- Na JY, Song K, Lee JW, et al. (2016) 6-Shogaol has anti-amyloidogenic activity and ameliorates Alzheimer’s disease via CysLT1R-mediated inhibition of cathepsin B. Biochem Biophys Res Commun. 477(1): 96-102. doi: 10.1016/j.bbrc.2016.06.026.
- Ho SC, Chang KS, Lin CC. (2013) Anti-neuroinflammatory capacity of fresh ginger is attributed mainly to 10-gingerol. Food Chem. 141(3): 3183-91. doi: 10.1016/j.foodchem.2013.06.010.
- Momtaz S, Hassani S, Khan F, et al. (2018) Cinnamon, a promising prospect towards Alzheimer’s disease. Pharmacol Res. 130: 241-258. doi: 10.1016/j.phrs.2017.12.011.
- Chakrabarti S, Jana M, Roy A, et al. (2018) Upregulation of suppressor of cytokine signaling 3 in microglia by cinnamic acid. Curr Alzheimer Res 15: 894–904.
- Kumar A, Konar A, Garg S, et al. (2021) Experimental evidence and mechanism of action of some popular neuro-nutraceutical herbs. Neurochem Int. 149: 105124. doi: 10.1016/j.neuint.2021.105124.
- Gupta M, Kaur G. (2019) Withania somnifera (L.) Dunal ameliorates neurodegeneration and cognitive impairments associated with systemic inflammation. BMC Complement Altern Med. 19(1): 217. doi: 10.1186/s12906-019-2635-0.
- Kaur T, Kaur G. (2017) Withania somnifera as a potential candidate to ameliorate high fat diet-induced anxiety and neuroinflammation. J Neuroinflammation. 14(1): 201. doi: 10.1186/s12974-017-0975-6.
- Nemetchek MD, Stierle AA, Stierle DB, Lurie DI. (2017) The Ayurvedic plant Bacopa monnieri inhibits inflammatory pathways in the brain. J Ethnopharmacol.197: 92-100. doi: 10.1016/j.jep.2016.07.073.
- Dubey T, Chinnathambi S. (2019) Brahmi (Bacopa monnieri): An ayurvedic herb against the Alzheimer’s disease. Arch Biochem Biophys. 676: 108153. doi: 10.1016/j.abb.2019.108153.
- Zandi N, Pazoki B, Momeni Roudsari N, et al. (2021) Prospects of Saffron and its Derivatives in Alzheimer’s Disease. Arch Iran Med. 24(3): 233-252. doi: 10.34172/aim.2021.35.
- Sowndhararajan K, Deepa P, Kim M, et al. (2018) An overview of neuroprotective and cognitive enhancement properties of lignans from Schisandra chinensis. Biomed Pharmacother. 97: 958-968. doi: 10.1016/j.biopha.2017.10.145.