Kevin Spelman looks at three cases of acute inflammation in allergies, also known as hypersensitivities, and their clinical resolution.
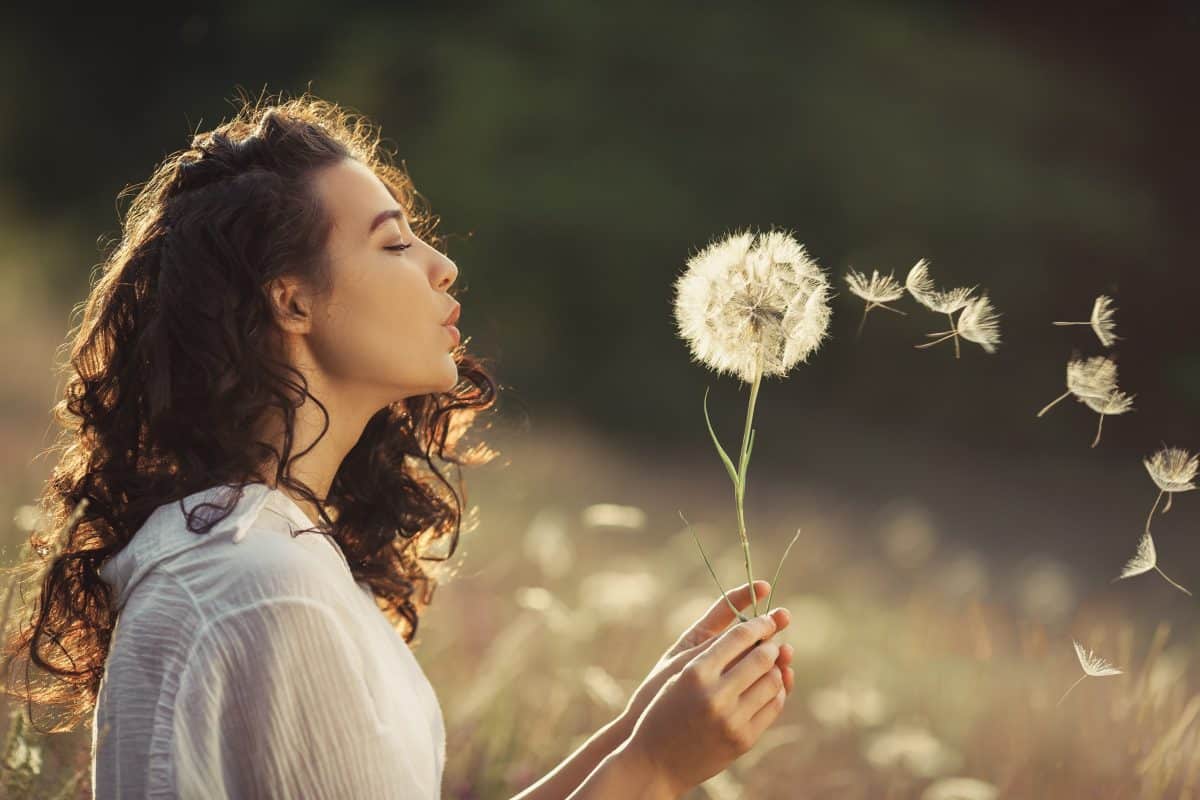
Inflammation is an evolutionarily conserved response characterized by the activation of immune and other somatic cells that provide protection from microorganisms, toxins and infections. This is typically characterized by the elimination of pathogens and the promotion of tissue repair and recovery (1,2).
When the process proceeds in a healthy body the process of inflammation is temporal restricted; it occurs when a threat is present and resolves once the threat has passed (3). Notably, the presence of select social, psychological, environmental and biological factors has been shown to inhibit the resolution of acute inflammation. This may result in a state of low-grade, non-infective systemic chronic inflammation characterized by the activation of immune components that are often distinct from those engaged during an acute immune response (4,5). For healthcare providers, it is key to understand both acute and chronic inflammatory processes.
The below perspective and collection of data come from my clinical experience of managing 3 different patients that responded quickly to what appeared to by an anaphylactic response to something in their diet. One case was directly observed in a classroom setting. Two other cases were done by phone as the patients were reacting to their allergens. Two other practitioners have shared case studies with the author that were successfully treated with Echinacea spp. and Taraxacum officinalis tincture for a total of just under a dozen cases that were postulated to be anaphylactic responses. Doses used are 5 ml of each extract every 20 minutes, for a total of 10 mL every 20 minutes until symptoms are resolved. In all three cases the dosing was done for a total of 3 doses.
Due to the focus on the previously mentioned clinical work this discussion will focus on the resolution of acute inflammation in hypersensitivities. The main pharmacological targets we will discuss are peroxisome proliferator-activated receptor gamma (PPARγ) and cannabinoid receptor type 2 (CB2). Keep in mind that both pharmacological sites below are also potentially useful for chronic inflammation.
Hypersensitivities
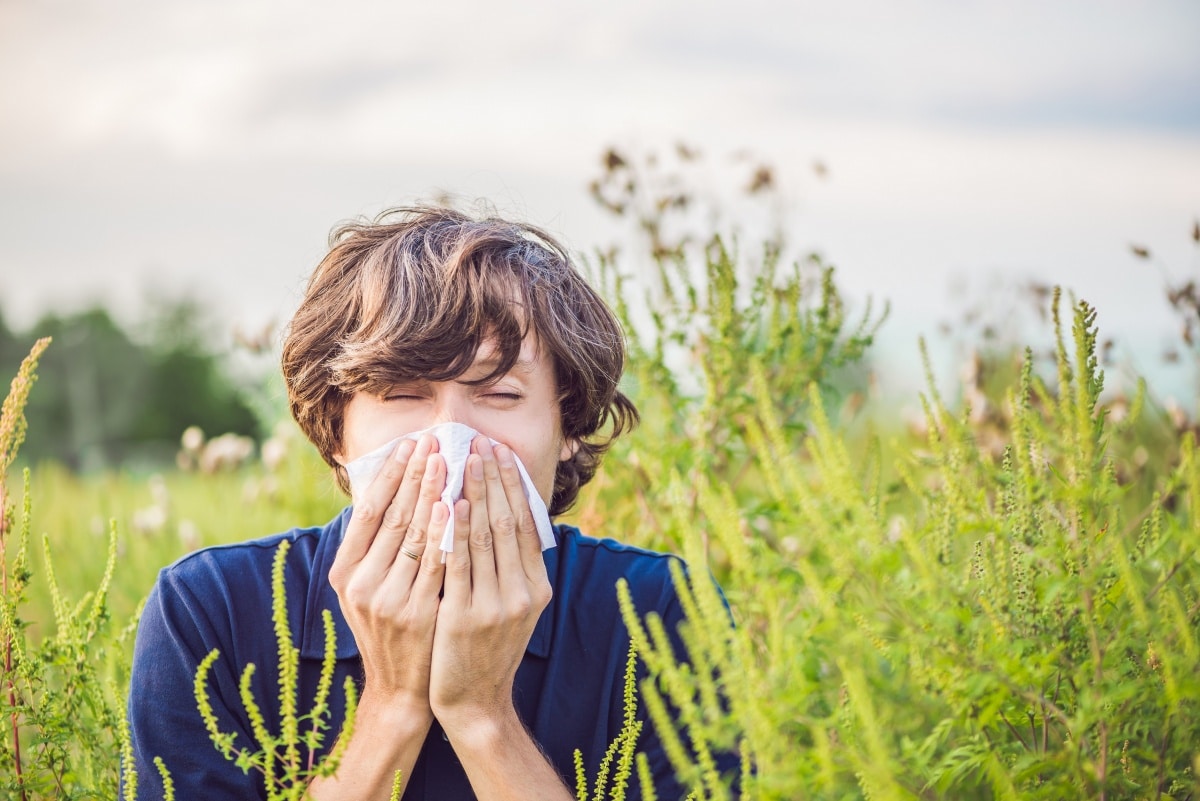
Allergies (also known as “hypersensitivities”) are due to overreactions of the immune system to substances that do not necessarily elicit immune responses in the majority of people. Hypersensitivities are grouped into four types; I, II, III and IV. These groupings are based on which white blood cells (WBCs) and tissues of the immune system are activated and how long it takes for a reaction to occur.
The two types of hypersensitivities that are referred to as allergies, are type I or ‘immediate’ hypersensitivities, and type IV or delayed type hypersensitivities (DTH).
The cells and pathways involved in type 1 hypersensitivities are mast cells, IgE and the release of histamine. An antigen induces the formation of IgE antibodies, in individuals with an atopic predisposition, via B lymphocytes. Reactions occur only after a requisite initial exposure, in which the primed mast cells bind IgE and start the degranulation process. This commonly involves the respiratory, gastrointestinal and/or the skin and occurs within minutes to hours.
In type IV hypersensitivities, or DTH, instead of involvement of the antibody IgE, T lymphocytes are involved and the onset of symptoms take hours to days to appear. CD4+ , T helper cells, in response to haptens (small antigens), release cytokines/chemokines which can recruit macrophages to a site (such as in the tuberculin-type response due to a TB test) inducing local inflammation. In the case of Type IV interactions the immune system does not need to be primed but does go through a sensitization phase. The outcome is acute inflammation and possibly edema. The skin is often the obvious site of DTH reactions (redness, swelling, hardening of the skin, rash, dermatitis).
The PPARγ receptor
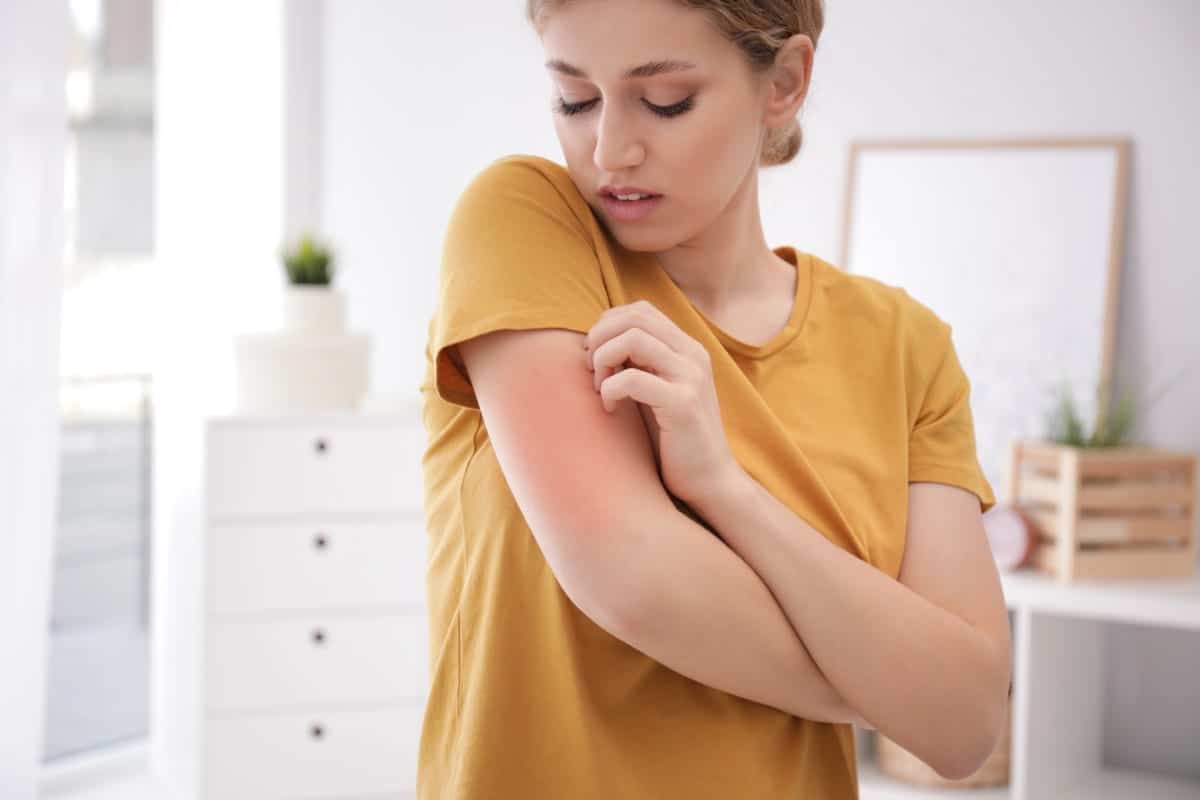
The PPARs constitute a set of three receptor sub-types which are members of the nuclear receptor superfamily of ligand-activated transcription factors. They are encoded by distinct genes that function as lipid sensors that regulate gene expression in many metabolically active tissues (6). The PPARs, so named because in early research it was found that PPAR-δ stimulation resulted in the increase in peroxisomes, have a significant role in cellular energy balance, fuel utilization, the metabolism of fatty acids and other lipids, the generation and remodeling of adipose tissue and fibrotic and hypertrophic responses in the heart and vascular wall. Many of these actions are via interactions with nuclear factors such as NFκB, NFAT and activator protein 1 (AP-1), thus modulating expression of pro-inflammatory cytokines and adhesion molecules, and altering cell signaling pathways (7,8). Thus, PPARs, in response to stressors, play a role in the complex orchestration of adaptive cellular physiology working in a concerted mode with the vitamin D receptor and the retinoic acid receptor (RXR). PPARs have been found to be involved in inflammation and carcinogenesis, and other immune activity. Thus, many pharmaceutical companies have been involved in PPAR research in search of the perfect ligand in areas such as diabetes, obesity, cardiovascular disease and immunology with drug development in mind.
The endogenous ligands to the PPAR sites were originally unknown, earning the PPARs the name “orphan nuclear receptors”. Key to this discussion, PPARγ plays a significant role in allergic response, specifically PPARγ (9-15). The high-affinity IgE receptor Fc epsilon RI (FcεRI), found on mast cells and basophils, plays a central role in IgE-mediated inflammatory reactions. PPARγ agonists have been shown to inhibit the expression of CD117 (tyrosine-protein kinase KIT or mast/stem cell growth factor receptor) and FcεRIα (9,11), and the maturation of bone marrow‑derived mast cells, as well as inhibiting the formation of granules and reducing the expression of β‑hexosaminidase (a marker of mast cell degranulation) and induce mast cell progenitor apoptosis (9). Finally a reduction in mast cell histamine content (15), histamine release (11,15) and suppression of body temperature increase in anaphylaxis models has been documented (12). Human trials of PPARγ agonist on patients with atopic dermatitis has demonstrated a decreased total body surface area involvement, severity of lesions, and number of flares with PPARγ agonists (16). In allergic asthmatic patients a down-regulation of PPARγ expression has been observed in lung tissue (17,18) and noted to be a potential factor in dysregulation of pulmonary homeostasis (17). While in steroid resistant asthmatic smokers an inhaled PPARγ agonist has shown improvements in lung function superior to steroid inhalation (19).
Human data in a month long double-blind randomized controlled trial, with a PPARγ agonist vs. placebo reduced the late asthmatic response by 15% and a reduction of exhaled nitric oxide by 14% (20). Another double blind RCT of a PPARγ agonist in severe asthmatic patients was found not to have a positive outcome (21). While PPARγ as a pharmacological target has strong preclinical data, the evidence in clinical trials is not robust. It may be that PPARγ needs concurrent support by engaging other pharmacological targets within a physiological network of allergic reactions. Intriguingly, PPARγ is now considered part of the endocannabinoid system (22-26).
The CB2 receptor
The CB2 receptor is a G protein-coupled receptor located on T & B lymphocytes, as well as natural killer cells, macrophages, neutrophils and mast cells (27). The CB2 receptor has been found to play a significant role in immune dynamics including the resolution of inflammation, cancer, atherosclerosis, osteoporosis and chronic pain (28). The CB2 expression in lymph nodes and spleen is higher than in peripheral blood cells and is different in various immune cell populations; B cells > NK cells > monocytes > neutrophils > CD8 T-cells > CD4 T-cells (29,30).
CB2 is a particular attractive for cannabinoid agonists selective for this site because of a paucity of psychomimetic activity. Nonetheless, in the face of remarkable volumes of preclinical data, only one CB2 potential drug molecule (cannabinor) has made it to phase II clinical trials (31). Other human data comes from marijuana smokers provide insight as to the effects of CB2 ligands on immune function. Lung alveolar macrophages removed from marijuana smokers have diminished capacity for the generation of TNF, gmCSF and IL-6 (inflammatory cytokines) (32).
Notably, CB2 ligands have demonstrated the inhibition of FcεRI-induced degranulation in mast cells in a concentration dependent manner. GPR55 (a putative CB3 receptor) also appears to show the same effect. CB1 appears not to have a role in inhibition of mast cell degranulation (33).
CB2 agonist have also shown a role in the retention of immature B cells in the bone marrow (34) and demonstrated a significant decrease in CXCR4 (a chemotactic chemokine) in bone marrow cells (34). CB2 is involved in the inhibition of lymphocyte recovery after bone marrow transplantation as well (35).
Other data suggest that cannabinoids can inhibit the production of TNF and other cytokines by several different pathways, some independent of cannabinoid receptors (27). Conversely, cannabinoids have also been shown to increase the production of cytokines (including TNF, IL-1, IL-6, and IL-10) if administered with appropriate immune stimulation (bacteria or antigens) (27) or, in some cases, without immune stimulation (36). Challenging a common misconception of CB2, this strongly suggest true immunomodulation and not simple immunosuppression.
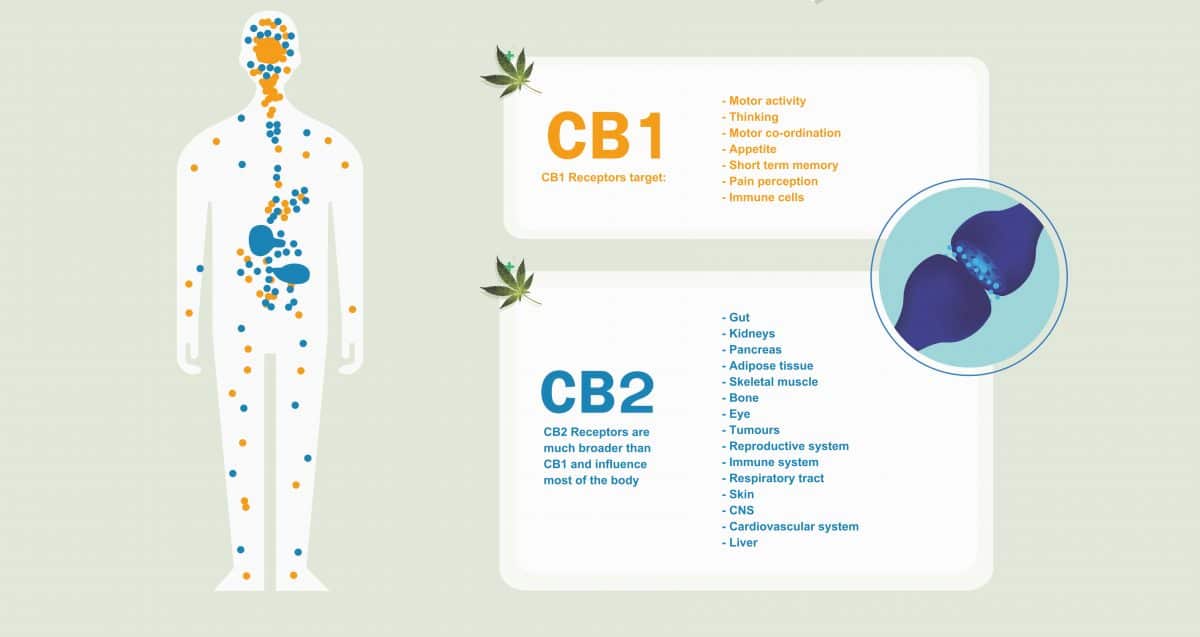
The herbs
The use of herbal remedies as a substitute for medical care is growing rapidly (37). An observation by this author is that in the United States, due to lack of s public health care system, every recession is accompanied by an increase in the sales of natural products. This is likely due to health insurance being tied to employment. As U.S. citizens lose their jobs, they also lose their health insurance.
Intriguingly, laboratory studies suggest that in some cases the overall pharmacological effects and therapeutic efficacies from medicinal plants may not derive from a single compound but from several compounds generating synergic activity (38-42). Synergy as a pharmacological construct, is often cited as a general mode for the biological activity of these compounds (38-43).
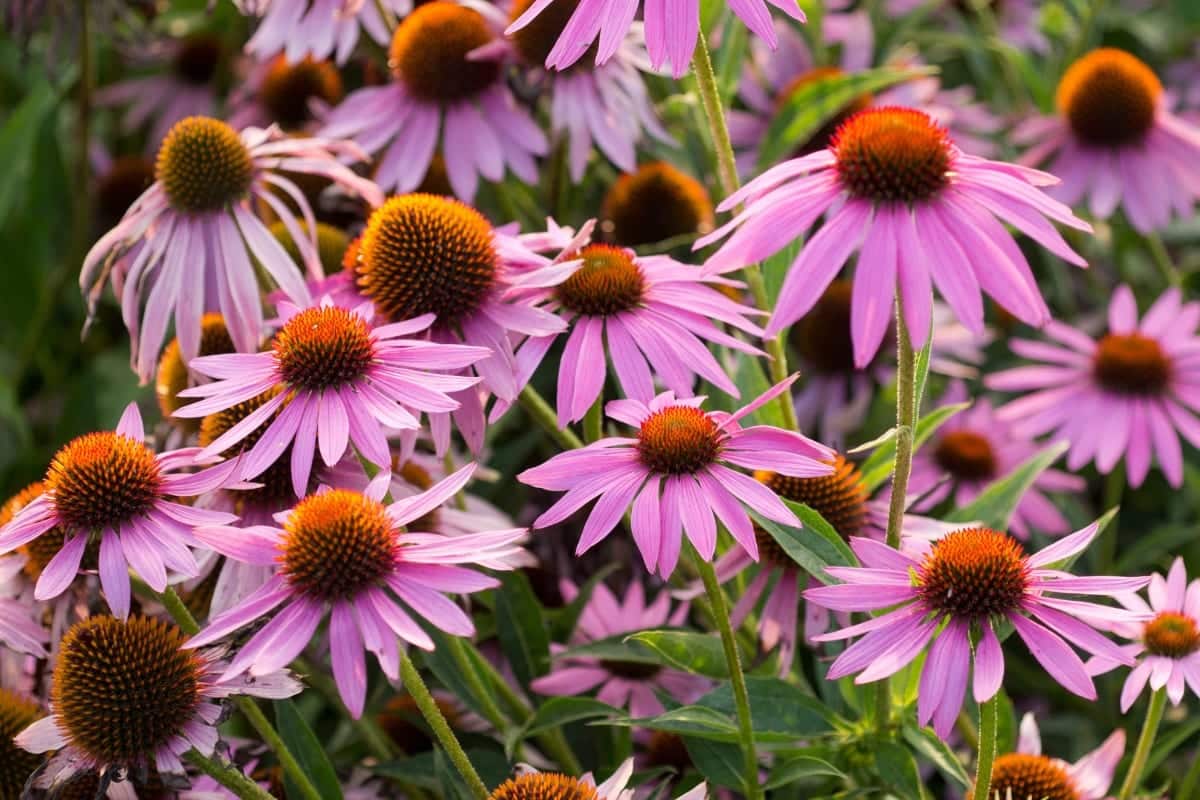
Preparations of Echinacea spp., have been used for more than a century for treatment of a variety of infections (44). More than 3 million physician prescriptions for Echinacea preparations are written annually in Germany (45) where there are more than 800 Echinacea products on the market (46). Most preparations are derived from the aerial parts of E. purpurea and underground parts of E. purpurea, E. angustifolia, or E. pallida (47).
Although controversial, the Echinacea spp. are often used interchangeably for the treatment of colds, flus, respiratory infections, and inflammations (48). Echinacea is documented to have immunostimulating, anti-viral, antibacterial, antifungal, insecticidal properties and anti-inflammatory properties (47,49).
Despite some negative outcomes in a few clinical trials using echinacea to treat upper respiratory infections, there are enough positive data in the human trials to offset the negative results. As a result, the meta-analyses that have been performed suggest that Echinacea products are effective (50-53). A Cochrane review reports some Echinacea preparations may be better than placebo and that the majority of the Echinacea studies demonstrate positive results (51). A meta-analysis by Schoop et al. (52), reports that standardized extracts of Echinacea were effective in the prevention of symptoms of the common cold as compared with placebo. Islam and Carter (53) conclude that there is a beneficial effect from Echinacea, but also suggest that differences in products and doses make evaluation challenging (51). Linde et al. (50) suggest that there is evidence, although inconsistent, that Echinacea is effective in treating URIs. A meta-analysis of studies with children (< 18 y/o) found that Echinacea reduces the incidence of URIs by 40% (54). Finally, the most recent meta-analysis finds that the evidence supports Echinacea’s benefit in decreasing the incidence and duration of the common cold in adults (55).
Echinacea’s effectiveness is believed to be related more to the enhancement of innate immunity, rather than antimicrobial activity (49,56) The stimulation of innate immunity by Echinacea extracts are well established (57-65). Studies have reported that echinacea extracts have the ability to activate human phagocytic function both in vitro and in vivo (58,59,61,66-68). Additionally, the plant has shown ex vivo immune stimulation in human immunodeficiency disorders (60). The immunomodulatory effects are believed to be mediated by induction of cytokines from macrophages and antioxidant activity (49,69). However, some people, mostly those who are not experienced with the clinical use of echinacea, believe that echinacea products are simple immunostimulating and as such, would be contraindicated in an attenuating an acute immune response. Only recently has basic research supported a biphasic response, clinicians who have extensive experience with echinacea preparations have been aware of this for many decades.
Echinacea spp. as immunomodulators
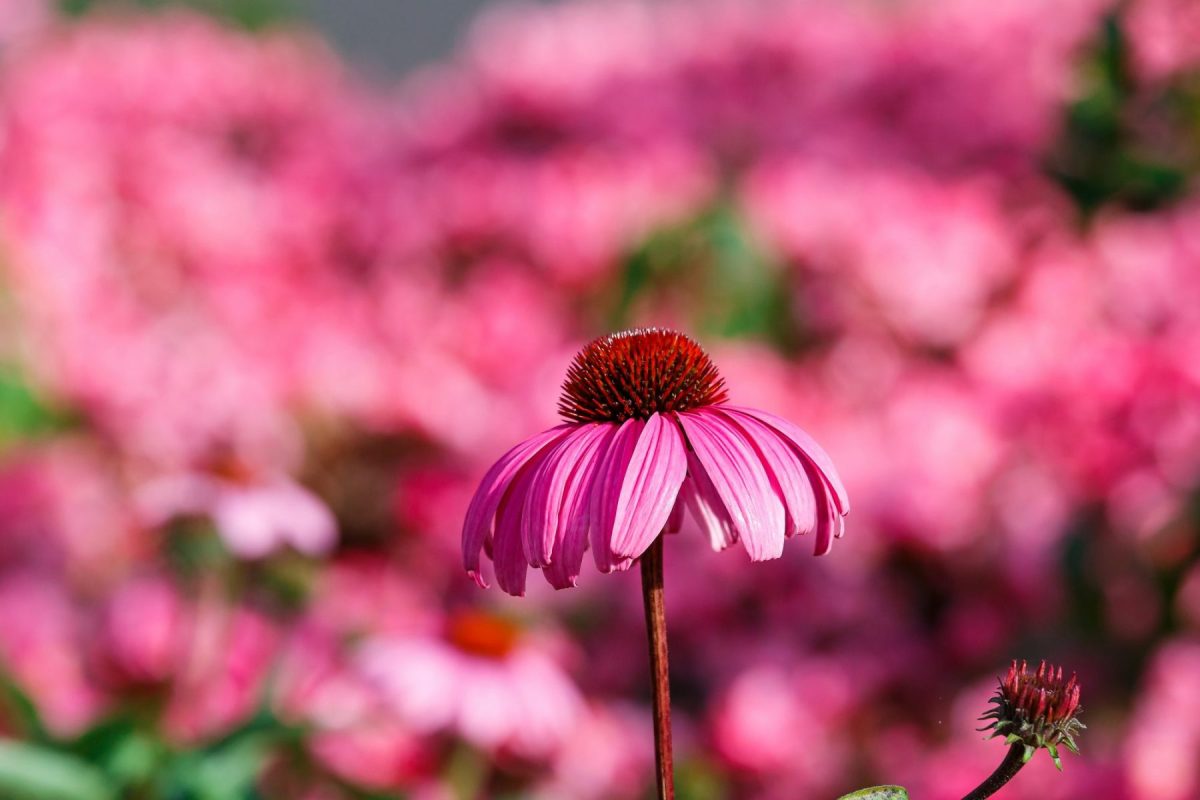
The search for endogenous ligands for the cannabinoid receptors has led to the discovery of several polyunsaturated compounds derived from fatty acids, mostly arachidonic acid (70). These fatty alkylamides, although not as widespread as other classes of natural products, are relatively abundant in plants, and occur in a variety of families, including the Asteraceae, Brassicaceae, Leguminosae, Piperaceae, and Rutaceae (71). Examples of plant species containing alkylamides similar to those of Echinacea spp. can be found in Spilanthes and Zanthoxylum species (28).
The alkylamides found in echinacea species and other medicinal plants, have been of pharmacological interest since humans first noted the tingling and numbing effect from chewing plants rich in these compounds (72). The anesthetizing tingling of these compounds is associated with activation of tactile and thermal trigeminal neurons (73). This property was utilized by native Americans (74) and eventually by physicians in the early 20th century for a variety of purposes including toothache and infections (75). Alkylamides were later recognized as insecticidal by a number of researchers (72,76-78) but eventually interest in these compounds waned. Nevertheless, these fatty acid derivatives have become a subject of renewed interest in the last few decades due to their recent identification as cannabinoid ligands (28,79-81).
The endogenous cannabinoid ligands are the fatty acid metabolites, known as eicosanoids (82). These endocannabinoids (e.g. anandamide from the Sanskrit term “ananda,” meaning bliss and 2-arachidonoyl glycerol) are structurally similar to the alkylamides, which are also fatty acid derivatives, and show nanomolar affinity for the type-2 cannabinoid receptor (CB2).
The affinity of the alkylamides for the CB2 are in the same range as the endogenous ligand anadamide (79). Research supports this, E. purpurea extracts demonstrate that particular isobutylamides modulate TNF mRNA expression in monocytes via CB2 receptors (79,81). Alkylamides binding to CB2 increased IL-8 and monocyte chemoattractant protein, both proinflammatory proteins (79). This may offer new insight into not only the mode of activity of Echinacea, but also immunology. Nadja Cech’s group, in collaboration with Bastyr University demonstrated a typical cannabinoid response from both extract and alkylamides of Echinacea; select isobutylamides from E. purpurea inhibited IL-2 expression in T-helper cells (83). Additionally, recent work demonstrates a TH-biasing effect, in which TH1-cell activity is suppressed and TH2-cell activity is increased (80). The cannabinoids show the same effect and this makes a case for the treatment of chronic inflammatory diseases by CB2 ligands (27).
Some have suggested since alkamides have anti-inflammatory activity, this is the primary effect of echinacea preparations (84). Anti-inflammatory activity has been verified through the inhibition of cyclooxygenase and 5-lipoxygenase (47) and the inhibition of IL-2 (83). Nonetheless, there is quite a collection of peer-reviewed research that shows immunostimulation by echinacea extracts and its compounds (57-68).
As for echinacea and PPAR activity, in the author’s hands, we observed inhibition of IL-2 via PPARγ activation (85). We also found that a large number of the alkylamides across the spectrum of unsaturation that differentiate this class of compounds activate PPAR (89).
Previous research has also demonstrated that Echinacea extracts inhibit mast cell degranulation. The authors of this research concluded that inhibition of mast cell activation coupled with the well-established inhibition of proinflammatory cytokine effects by alkylamide rich Echinacea extracts suggest utility in allergic diseases (90).
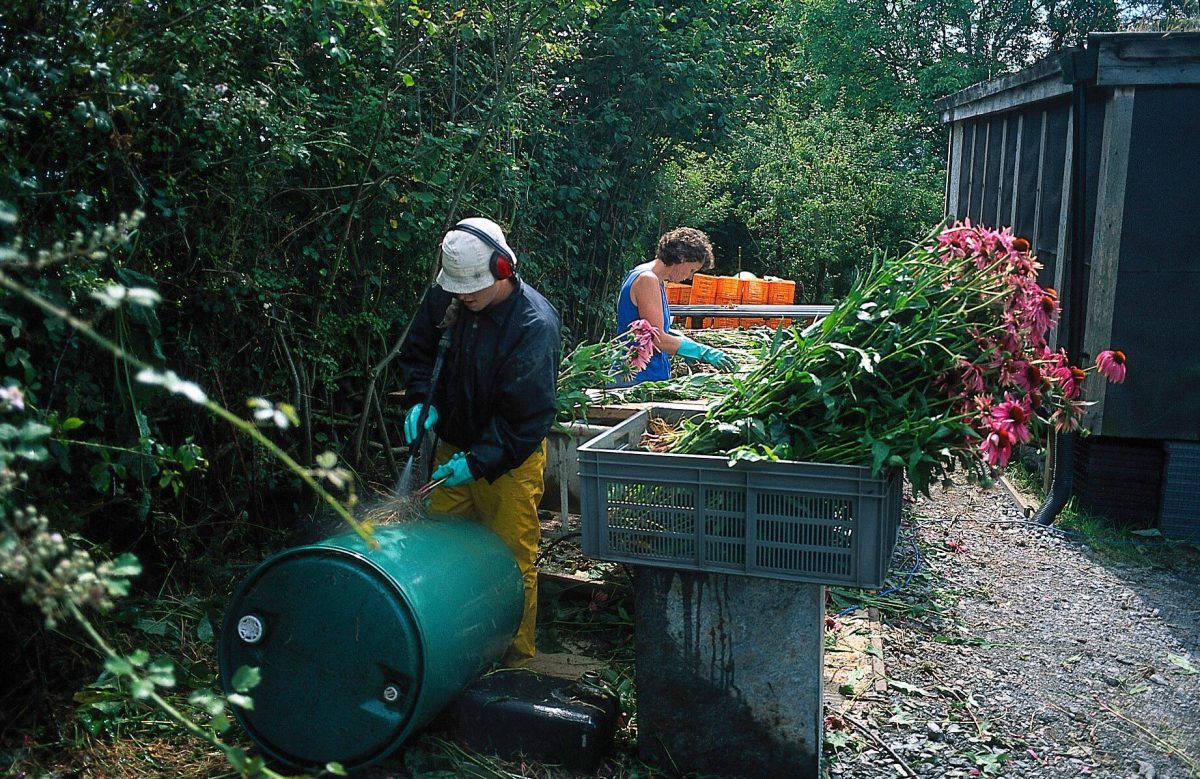
The alkylamides are not the only active constituents. There are other compounds in the Echinacea spp. that deserve mention. Three other constituent groups have shown activity; the hydroxycinnamates (caftaric acid, caffeic acid, chlorogenic acid, cichoric acid, cinnamic acid, cynarin, echinacoside etc.), polysaccharides, and glycoproteins (91,92).
Another phytocompound found in Echinacea, as well as cinnamon, clove, rosemary and black pepper, and such medicinal plants as Bidens pilosa, Angelica archangelica and Apium graveolens, isβ-caryophyllene (BCP), a sesquiterpene. BCP is considered a phytocannabinoid as it binds CB2. Research shows that via a CB2 mode of activity BCP has demonstrated significant cardioprotective effects in preclinical research in induced myocardial infarction models. Notably, BCP reduced the myocardial expression of inflammasome proteins including NLRP3, procaspase-1 and pro-IL-1β via TLR4 mediated NF-κB/MAPK signaling in CB2 dependent manner. Moreover, BCP enhanced the expression of the CB2 receptor and PPARγ and activated myocardial CB2 receptors resulting in the mitigation of oxidative injury (93).
Considering the number of phytochemical families that are active, the explanations of the effect of Echinacea include suggestions that the activity may be due to an entourage effect (85). There has also been in vitro antioxidant activity demonstrated by the hydroxycinnamates for both E. purpurea and E. angustifolia extracts (94,95). However, antioxidant activity will be addressed in the next section on Taraxacum officinale.
Taraxacum officinale
One of the most ubiquitous medicinal plants, Dandelion (Taraxacum officinale) is well-known as a food due to its rich content in nutrients. Although considered by many to be an annoying weed, every traditional culture that has a native Taraxacum species has found medicinal use for this versatile genera (97). Unfortunately, dandelion is often overlooked due to is prevalence. An initial clue into its medicinal properties is provided by the name Taraxacum which is derived from the Greek words “taraxis” for inflammation and “akeomai” for curative (98). Moreman (74) reviews use of dandelion by various tribes of the native North American culture shows use various parts of the dandelion for food and medicine. For example, the spring stems and leaves were used as vegetables and eaten to build the blood for conditions such as anemia and as a laxative-tonic, while decoctions of the roots were taken for stomach pain and to produce postpartum milk flow. Infusions of leaf and root were taken for kidney trouble and dropsy and used as a bitter tonic, the flower blossoms were taken for menstrual cramps.
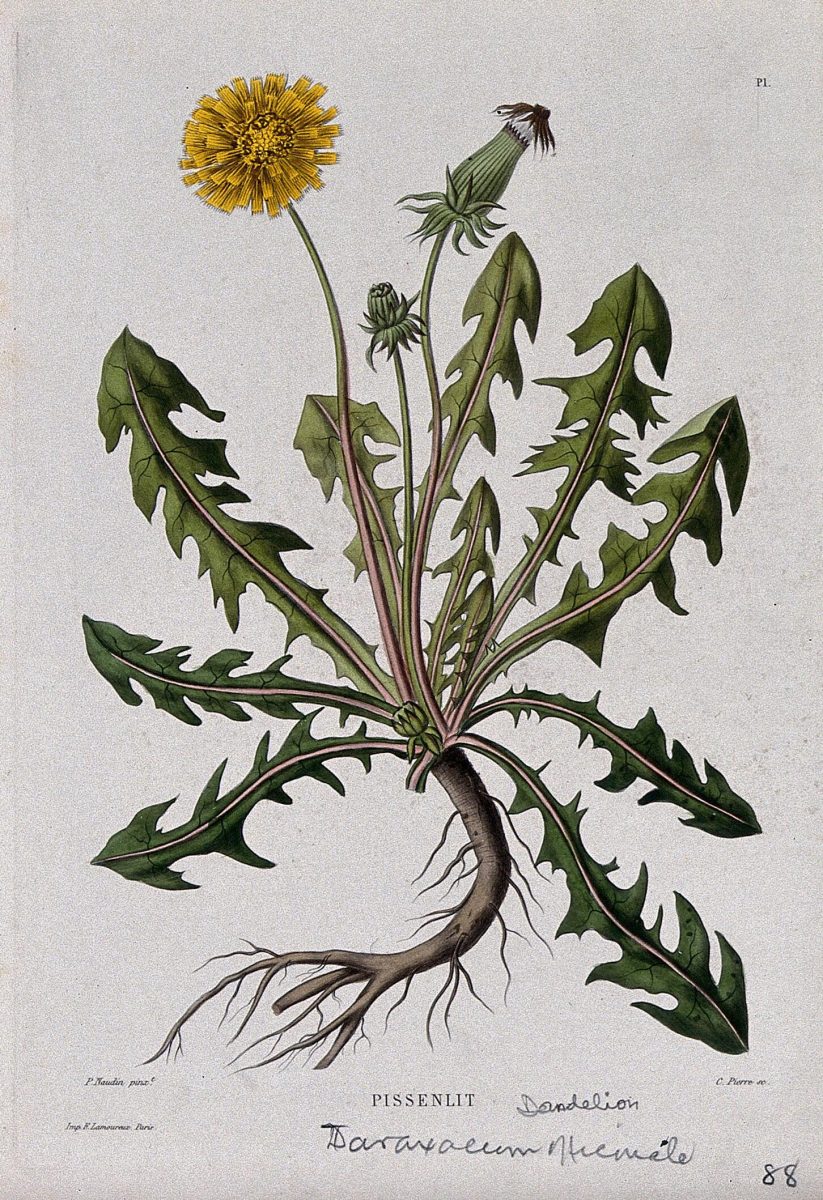
In traditional Chinese medicine Taraxacum mongolicum is thought to have activity in a variety of health benefits (99), this includes clearing heat, especially of the liver and urinary tract and to promote lactation (100). In traditional Ayurvedic medicine T. officinale is used similarly as in TCM and said to be valuable in liver disorders and a useful diuretic (101) and to clear heat (102). An early 19th century reference suggests that Taraxacum was used for chronic inflammation, digestive problems, as well as cirrhosis (103).
Although the diuretic properties are well known and recently observed in the first human trial (104), this brief overview will focus on the immune properties of dandelion.
The anti-inflammatory activity of dandelion is most likely partially due to its antioxidant activity and its hepatic activity. Recall that the liver is a key lymphatic organ with the largest population of fixed macrophages (Kupffer cells) of any bodily tissue and is therefore influential on the reticuloendothelial system and immune response. Much of the traditional use of dandelion has supported hepatic activity for dandelion, indications such as liver and/or gall-bladder inflammation and stasis, cholelithiasis, metabolic toxicity, jaundice, hepatitis, and dyspepsia secondary to deficient bile secretion (105). Medicinal plant preparations in general that have hepatic activity are likely to also influence Kupffer cells, although in varying degrees. This obviously can lead to immune up or down regulation. As a hepatic anti-inflammatory, dandelion, at least in clinical observations, appears to also have a systemic anti-inflammatory effect. Phenolic compounds are believed to provide some of the anti-inflammatory activity (98).
A large portion of the research on the activity of dandelion has focused on the antioxidant activity (99,106-109). Past research reports significant antioxidant activity, typical for polyphenol rich plants, and inhibition of inducible nitric oxide synthase (iNOS), as well as synergic activity with other micro and phyto-nutrients such as the ubiquitous catechins and ascorbate (99,106,107). Sumanth and Rana (108) confirmed an antioxidant effect and observed increases in the levels of superoxide dismutase, catalase and glutathione using a hydroethanolic preparation. Zhu et al (109) using a hydroethanolic extract, confirmed Rana’s results in finding increased superoxide dismutase, catalase, glutathione levels along with peroxidase levels and also found reduced lipid peroxidation. Hu and Kitts (99) demonstrated protection of cells from peroxyl-radical-induced intracellular oxidation speculating that the protection may have been the result of scavenging of intercellular and intracellular peroxyl radicals by a phenolic rich extract from dandelion flowers. This team also showed a synergic effect with α-tocopherol and a 40% ascorbate equivalence which they believed was responsible in regenerating the α-tocopherol. Thus, dandelion’s antioxidant activity is established.
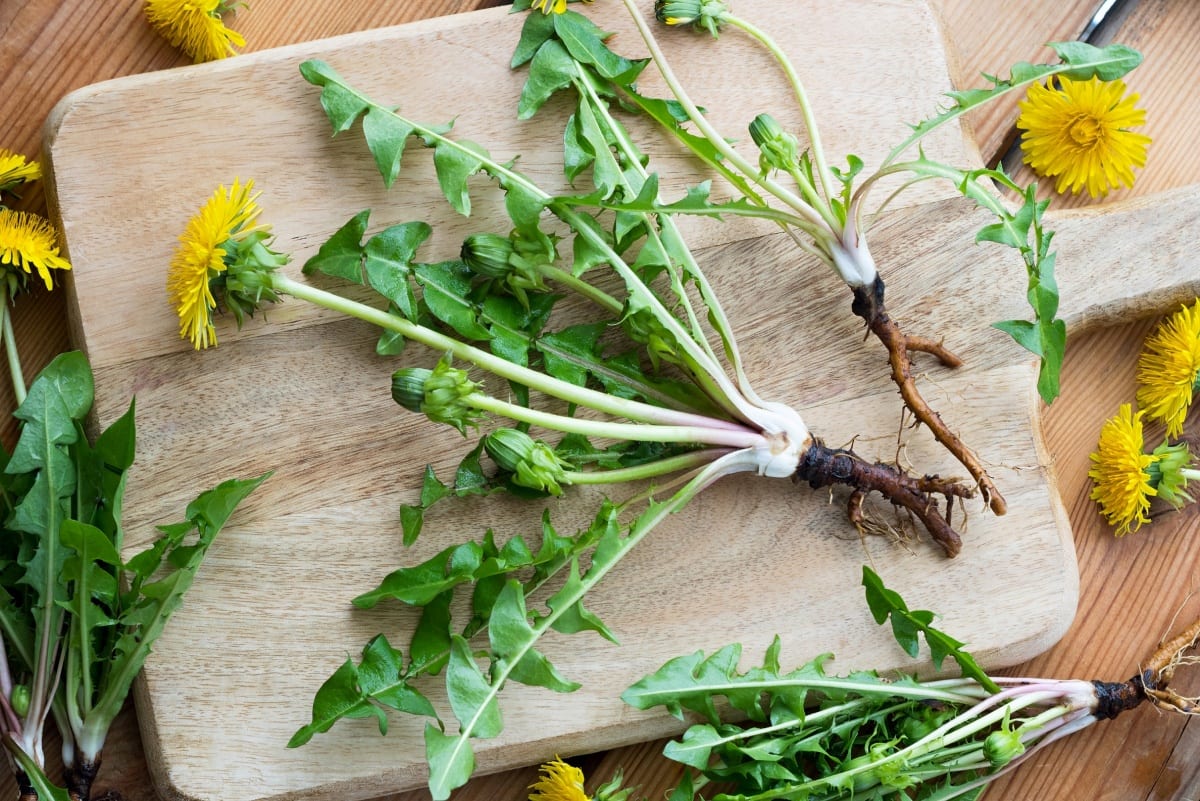
Antioxidant activity has generally been accepted to be a mode of activity for immunomodulation (110-112). Both anti-inflammatory and antiallergic properties are known to be induced be particular antioxidants (113). The reduction/oxidation (redox) reactions that are crucial to immune function can lead to tissue damage, allergy and autoimmune diseases if balance in this system is lost. Research has confirmed that polyphenols can make improvements in the overall redox status of immune cells (114). In compromised subjects (aged or diseased) this can lead to enhancements in such functions as chemotaxis capacity, microbicidal activity, lymphoproliferative response to mitogens, interleukin-2 (IL-2) and tumor necrosis factor (TNF alpha) release (111). For example, in preclinical (in vivo) models many of the gallate derivatives have exhibited inhibition of histamine release from mast cells (murine model) (112). Additionally, the catechins are known to significantly inhibit type IV allergies in murine models (110). Finally, kaempferol has shown positive effects on IL-4 and JAK3-dependent responses suggesting that compounds rich in this compound may have positive influence on allergies, autoimmune conditions and cancer (115).
Another pathway where dandelion has exhibited activity is in the nitric oxide (NO) pathway. NO is an important effector molecule that plays a role in the majority of the steps of inflammation, and if unchecked, can also lead to tissue damage, inflammation and is involved in allergic response (116). Taraxacum extracts and individual constituents have demonstrated inhibition of inducible nitric oxide from immune cells (99,117).
Considerations of medicinal plant medicines
It must be kept in mind that many of these effects are based on in vitro and in vivo preclinical research. Regardless, the bottom line is that in the previously mentioned cases the treatments with echinacea and dandelion were effective. From an energetic model of medicine many of the pathways discussed can be seen as leading to a reduction in the “heat” of a system. That is, the inflammatory process is interrupted or reduced and therefore the overall effect of this process can be seen as “cooling” the inflammatory processes. Considering the combination of E. purpurea extracts and T. officinale extracts we have multiple modes of activity including affecting key messenger molecules (see Table 1). The alkylamides via CB2 may down regulate cytokine production. Additionally, the inhibition of cyclooxygenase and lipoxygenase, as well as antioxidant activity, are likely responsible for the effects in the patients discussed above. Hydroxycinnamates, present in both plants, especially cichoric acid and caffeic acid are some of the most efficient antioxidants from natural sources (118). Additionally, direct activity on mast cell degranulation is a strong possiblity, considering the modes of activity of CB2 and PPARγ and the speed in which these patients recovered from an acute hypersensitivity.
An interesting thought lies in the potential of the improvement in other tissues and biochemical pathways influencing hypersensitivities that extracts of T. officinale radix and E. purpurea radix may have. In other words, this likely served as more than a treatment for downregulating an immune process. Rather, there were likely beneficial effects related to other activities, and other undiscussed constituents of the plants that are still unseen. As the late, great herbalist Michael Moore once said “the advantage of medicinal plants is that they are broad spectrum and naturally dilute. The disadvantage of medicinal plants is that they are broad spectrum and naturally dilute.”
CB2 ligands | PPARγ ligands |
---|---|
1. Anandamide MW 347.3 | 6. 15-deoxy-Δ (12,14)-prostaglandin J2 MW 316.4 (fibroblasts activity 7 μM) |
2. 2-arachidonylglycerol MW 378.3 (PPARγ) | 7. 13-hydroxyoctadecadienoic acid MW 296.4 |
3. Dodeca-2E,4E,8Z,10Z-tetraenoic acid isobutylamide MW 247.3 from Echinacea spp. | 8. 13- oxooctadecadienoic acid MW 294.4 |
4. Dodeca-2E, 4E-ene-dienoic acid isobutylamide MW 251.3 from Echinacea spp. | 9. Undeca-2E-ene-8,10-diynoic acid isobutylamide MW 231.3 from Echinacea spp. |
5. Dodeca-2E,4E,8Z-trienoic acid isobutylamide MW 249.3 from Echinacea spp. | 10. Hexadeca-2E,9Z,12Z,14E-tetraenoic acid isobutylamide MW 303.3 from Echinacea spp. |
b. Compounds 3 & 4 are also PPARγ activators. from 87with Ki of 57 nM and 60 nM (88)
References
- Netea MG, Balkwill F, Chonchol M, et al. A guiding map for inflammation. Nat Immunol. 2017;18(8):826-831.
- Furman D, Campisi J, Verdin E, et al. Chronic inflammation in the etiology of disease across the life span. Nat Med. 2019;25(12):1822-1832.
- Kotas ME, Medzhitov R. Homeostasis, inflammation, and disease susceptibility. Cell. 2015;160(5):816-827.
- Straub RH. The brain and immune system prompt energy shortage in chronic inflammation and ageing. Nat Rev Rheumatol. 2017;13(12):743-751.
- Calder PC, Ahluwalia N, Albers R, et al. A consideration of biomarkers to be used for evaluation of inflammation in human nutritional studies. Br J Nutr. 2013;109 Suppl 1:S1-34.
- Bishop-Bailey D, Wray J. Peroxisome proliferator-activated receptors: a critical review on endogenous pathways for ligand generation. Prostaglandins Other Lipid Mediat. 2003;71(1-2):1-22.
- Touyz RM, Schifffrin EL. Peroxisome proliferator-activated receptors in vascular biology-molecular mechanisms and clinical implications. Vascul Pharmacol. 2006;45(1):19-28.
- Chung SW, Kang BY, Kim TS. Inhibition of interleukin-4 production in CD4(+) T cells by peroxisome proliferator-activated receptor-gamma (PPAR-gamma) ligands: Involvement of physical association between PPAR-gamma and the nuclear factor of activated T cells transcription factor. Mol Pharmacol. 2003;64(5):1169-1179.
- Zhang Y, Li X, Fang S, et al. Peroxisome proliferator-activated receptor γ agonist suppresses mast cell maturation and induces apoptosis. Mol Med Rep. 2017;16(2):1793-1800.
- Zhao CC, Xu J, Xie QM, Zhang HY, Fei GH, Wu HM. Abscisic acid suppresses the activation of NLRP3 inflammasome and oxidative stress in murine allergic airway inflammation. Phytother Res. 2021;35(6):3298-3309.
- Fujimura Y, Tachibana H, Yamada K. Peroxisome proliferator-activated receptor ligands negatively regulate the expression of the high-affinity IgE receptor Fc epsilon RI in human basophilic KU812 cells. Biochem Biophys Res Commun. 2002;297(2):193-201.
- Nagata K, Kasakura K, Miura R, Yashiro T, Nishiyama C. Suppressive role of PPARγ in the IgE-dependent activation of mast cells. Int Immunol. 2020;32(2):143-150.
- Seo SW, Koo HN, An HJ, et al. Taraxacum officinale protects against cholecystokinin-induced acute pancreatitis in rats. World J Gastroenterol. 2005;11(4):597-599.
- Wang J, Guo X, Chen C, et al. Gender differences in food allergy depend on the PPAR γ/NF-κB in the intestines of mice. Life Sci. 2021;278:119606.
- Tachibana M, Wada K, Katayama K, et al. Activation of peroxisome proliferator-activated receptor gamma suppresses mast cell maturation involved in allergic diseases. Allergy. 2008;63(9):1136-1147.
- Behshad R, Cooper KD, Korman NJ. A retrospective case series review of the peroxisome proliferator-activated receptor ligand rosiglitazone in the treatment of atopic dermatitis. Archives of dermatology. 2008;144(1):84-88.
- Liu J, Sakurai R, Rehan VK. PPAR-γ agonist rosiglitazone reverses perinatal nicotine exposure-induced asthma in rat offspring. Am J Physiol Lung Cell Mol Physiol. 2015;308(8):L788-796.
- Lotfi R, Davoodi A, Mortazavi SH, et al. Imbalanced serum levels of resolvin E1 (RvE1) and leukotriene B4 (LTB4) in patients with allergic rhinitis. Mol Biol Rep. 2020;47(10):7745-7754.
- Spears M, Donnelly I, Jolly L, et al. Bronchodilatory effect of the PPAR-gamma agonist rosiglitazone in smokers with asthma. Clin Pharmacol Ther. 2009;86(1):49-53.
- Richards DB, Bareille P, Lindo EL, Quinn D, Farrow SN. Treatment with a peroxisomal proliferator activated receptor gamma agonist has a modest effect in the allergen challenge model in asthma: a randomised controlled trial. Respir Med. 2010;104(5):668-674.
- Kaler M, Barochia AV, Weir NA, et al. A randomized, placebo-controlled, double-blinded, crossover trial of pioglitazone for severe asthma. J Allergy Clin Immunol. 2017;140(6):1716-1718.
- Rockwell CE, Kaminski NE. A cyclooxygenase metabolite of anandamide causes inhibition of interleukin-2 secretion in murine splenocytes. J Pharmacol Exp Ther. 2004;311(2):683-690.
- Rockwell CE, Snider NT, Thompson JT, Vanden Heuvel JP, Kaminski NE. Interleukin-2 suppression by 2-arachidonyl glycerol is mediated through peroxisome proliferator-activated receptor gamma independently of cannabinoid receptors 1 and 2. Mol Pharmacol. 2006;70(1):101-111.
- Lenman A, Fowler CJ. Interaction of ligands for the peroxisome proliferator-activated receptor gamma with the endocannabinoid system. Br J Pharmacol. 2007;151(8):1343-1351.
- O’Sullivan SE. Cannabinoids go nuclear: evidence for activation of peroxisome proliferator-activated receptors. Br J Pharmacol. 2007;152(5):576-582.
- Pistis M, Melis M. From surface to nuclear receptors: the endocannabinoid family extends its assets. Curr Med Chem. 2010;17(14):1450-1467.
- Klein TW. Cannabinoid-based drugs as anti-inflammatory therapeutics. Nat Rev Immunol. 2005;5(5):400-411.
- Gertsch J, Raduner S, Altmann KH. New natural noncannabinoid ligands for cannabinoid type-2 (CB2) receptors. J Recept Signal Transduct Res. 2006;26(5-6):709-730.
- Galiègue S, Mary S, Marchand J, et al. Expression of central and peripheral cannabinoid receptors in human immune tissues and leukocyte subpopulations. Eur J Biochem. 1995;232(1):54-61.
- Almogi-Hazan O, Or R. Cannabis, the Endocannabinoid System and Immunity-the Journey from the Bedside to the Bench and Back. Int J Mol Sci. 2020;21(12).
- Nevalainen T. Recent development of CB2 selective and peripheral CB1/CB2 cannabinoid receptor ligands. Curr Med Chem. 2014;21(2):187-203.
- Baldwin GC, Tashkin DP, Buckley DM, Park AN, Dubinett SM, Roth MD. Marijuana and cocaine impair alveolar macrophage function and cytokine production. Am J Respir Crit Care Med. 1997;156(5):1606-1613.
- Cruz SL, Sánchez-Miranda E, Castillo-Arellano JI, Cervantes-Villagrana RD, Ibarra-Sánchez A, González-Espinosa C. Anandamide inhibits FcεRI-dependent degranulation and cytokine synthesis in mast cells through CB(2) and GPR55 receptor activation. Possible involvement of CB(2)-GPR55 heteromers. Int Immunopharmacol. 2018;64:298-307.
- Pereira JP, An J, Xu Y, Huang Y, Cyster JG. Cannabinoid receptor 2 mediates the retention of immature B cells in bone marrow sinusoids. Nat Immunol. 2009;10(4):403-411.
- Khuja I, Yekhtin Z, Or R, Almogi-Hazan O. Cannabinoids Reduce Inflammation but Inhibit Lymphocyte Recovery in Murine Models of Bone Marrow Transplantation. Int J Mol Sci. 2019;20(3).
- Kishimoto S, Kobayashi Y, Oka S, Gokoh M, Waku K, Sugiura T. 2-arachidonoylglycerol, an endogenous cannabinoid receptor ligand, induces accelerated production of chemokines in HL-60 cells. J Biochem. 2004;135(4):517-524.
- Sharples FM, van Haselen R, Fisher P. NHS patients’ perspective on complementary medicine: a survey. Complement Ther Med. 2003;11(4):243-248.
- Spelman K, Duke JA, Bogenschutz-Godwin MJ. The Synergy Principle in Plants, Pathogens, Insects, Herbivores and Humans. In: Kaufman PB, ed. Natural products from plants. Vol Publication Forthcoming. Boca Raton, Fla.: CRC Press; 2005.
- Spelman K. Philosophy in Phytopharmacology: Ockham’s Razor vs. Synergy. J Herbal Pharmacotherapy. 2005;5(2):31-47.
- Wagner H. Phytomedicine Research in Germany. Environ Health Perspect. 1999;107:779-781.
- Williamson EM. Synergy and other interactions in phytomedicines. Phytomedicine. 2001;8(5):401-409.
- Spinella M. The importance of pharmacological synergy in psychoactive herbal medicines. Altern Med Rev. 2002;7(2):130-137.
- Wills RBH, Bone K, Morgan M. Herbal products: active constituents, modes of action and quality control. Nutrition Research Reviews. 2000;13(1):47-77.
- Couch JF, Giltner LT. An Experimental Study of Echinacea Therapy. J Agric Res. 1920;20(1):63-84.
- Barrett B, Vohmann M, Calabrese C. Echinacea for upper respiratory infection. J Fam Pract. 1999;48(8):628-635.
- Sun L, Rezaei KA, Temelli F, Ooraikul B. Supercritical fluid extraction of alkylamides from Echinacea angustifolia. J Agric Food Chem. 2002;50(14):3947-3953.
- Kim HO, Durance TD, Scaman CH, Kitts DD. Retention of alkamides in dried Echinacea purpurea. J Agric Food Chem. 2000;48(9):4187-4192.
- Bauer R, Foster S. Analysis of alkamides and caffeic acid derivatives from Echinacea simulata and E. paradoxa roots. Planta Med. 1991;57(5):447-449.
- Mills S, Bone K. Principles and Practice of Phytotherapy. New York: Churchill Livingston; 2000.
- Linde K, Barrett B, Wolkart K, Bauer R, Melchart D. Echinacea for preventing and treating the common cold. Cochrane Database Syst Rev. 2006(1):CD000530.
- Melchart D, Linde K, Fischer P, Kaesmayr J. Echinacea for preventing and treating the common cold. Cochrane Database Syst Rev. 2000;2(CD000530).
- Schoop R, Klein P, Suter A, Johnston SL. Echinacea in the prevention of induced rhinovirus colds: A meta-analysis. Clin Therap. 2006;28(2):174-183.
- Islam J, Carter R. Use of Echinacea in upper respiratory tract infection. South Med J. 2005;98(3):311-318.
- Del-Rio-Navarro BE, Espinosa RF, Flenady V, Sienra-Monge JJL. Immunostimulants for preventing respiratory tract infection in children. Cochrane Database Syst Rev. 2006;4(CD004974):DOI:10.1002/14651858.CD14004974.pub14651852.
- Shah SA, Sander S, White CM, Rinaldi M, Coleman CI. Evaluation of echinacea for the prevention and treatment of the common cold: a meta-analysis. The Lancet infectious diseases. 2007;7(7):473-480.
- Bauer R, Netsch M, Kreuter MH. Echinacea purpurea. Published 2005. Accessed May 11, 2005.
- Sun LZ, Currier NL, Miller SC. The American coneflower: a prophylactic role involving nonspecific immunity. J Altern Complement Med. 1999;5(5):437-446.
- Burger RA, Torres AR, Warren RP, Caldwell VD, Hughes BG. Echinacea-induced cytokine production by human macrophages. Int J Immunopharmacol. 1997;19(7):371-379.
- Melchart D, Linde K, Worku F, et al. Results of five randomized studies on the immunomodulatory activity of preparations of Echinacea. J Altern Complement Med. 1995;1(2):145-160.
- See DM, Broumand N, Sahl L, Tilles JG. In vitro effects of echinacea and ginseng on natural killer and antibody-dependent cell cytotoxicity in healthy subjects and chronic fatigue syndrome or acquired immunodeficiency syndrome patients. Immunopharmacology. 1997;35(3):229-235.
- Morazzoni P, Cristoni A, Di Pierro F, et al. In vitro and in vivo immune stimulating effects of a new standardized Echinacea angustifolia root extract (Polinacea). Fitoterapia. 2005;76(5):401-411.
- Goel V, Lovlin R, Chang C, et al. A proprietary extract from the echinacea plant (Echinacea purpurea) enhances systemic immune response during a common cold. Phytother Res. 2005;19(8):689-694.
- Goel V, Chang C, Slama JV, et al. Alkylamides of Echinacea purpurea stimulate alveolar macrophage function in normal rats. Int Immunopharmacol. 2002;2(2-3):381-387.
- Goel V, Chang C, Slama J, et al. Echinacea stimulates macrophage function in the lung and spleen of normal rats. J Nutr Biochem. 2002;13(8):487.
- Maass N, Bauer J, Paulicks BR, Bohmer BM, Roth-Maier DA. Efficiency of Echinacea purpurea on performance and immune status in pigs. J Anim Physiol Anim Nutr (Berl). 2005;89(7-8):244-252.
- Percival SS. Use of echinacea in medicine. Biochem Pharmacol. 2000;60(2):155-158.
- Roesler J, Steinmuller C, Kiderlen A, Emmendorffer A, Wagner H, Lohmann-Matthes ML. Application of purified polysaccharides from cell cultures of the plant Echinacea purpurea to mice mediates protection against systemic infections with Listeria monocytogenes and Candida albicans. Int J Immunopharmacol. 1991;13(1):27-37.
- Wilasrusmee C, Siddiqui J, Bruch D, Wilasrusmee S, Kittur S, Kittur DS. In vitro immunomodulatory effects of herbal products. Am Surg. 2002;68(10):860-864.
- Bauer R. Chemistry, analysis and immunological investigations of Echinacea phytopharma-ceuticals. In: Wagner H, ed. Immunomodulatory Agents from Plants. Basel: Birkhäuser Verlag; 1999:41-88.
- Kogan NM, Mechoulam R. The chemistry of endocannabinoids. J Endocrinol Invest 2006;29:3-14.
- Chapman KD. Occurrence, metabolism, and prospective functions of N-acylethanolamines in plants. Prog Lipid Res. 2004;43(4):302-327.
- Greger H. Alkamides: structural relationships, distribution and biological activity. Planta Med. 1984;50(5):366-375.
- Bryant BP, Mezine I. Alkylamides that produce tingling paresthesia activate tactile and thermal trigeminal neurons. Brain Res. 1999;842(2):452.
- Moerman DE. Native American ethnobotany. Portland, Or.: Timber Press; 1998.
- Ellingwood F. History, Character and Physiological Action. Ellingwood’s Therapeutist. 1917;11(Feb):4-6.
- Towers GHN, Champagne DE. Medicinal phytochemistry of the Compositae: The activites of selected acetylenes and their sulfur derivatives. In: Lam J, Breteler H, Arnason T, Hansen L, eds. Chemistry and biology of naturally-occurring acetylenes and related compounds (NOARC). Vol v7. Amersterdam; New York: Elsevier; 1988:139-178.
- Jacobson M. Structure of echinacein, the insecticidal component of American coneflower roots. J Org Chem. 1967;32(5):1646-1647.
- Jacobson M. Herculin, A Pungent Insecticidal Constituent of Southern Prickly Ash Bark. J Am Chem Soc. 948;70:4234.
- Woelkart K, Xu W, Pei Y, Makriyannis A, Picone RP, Bauer R. The Endocannabinoid System as a Target for Alkamides from Echinacea angustifolia Roots. Planta Med. 2005;71(8):701-705.
- Raduner S, Majewska A, Chen J-Z, et al. Alkylamides from Echinacea Are a New Class of Cannabinomimetics: Cannabinoid Type 2 Receptor-Dependent and -Independent Immunomodulatory Effects J Biol Chem. 2006;281(20):14192-14206.
- Gertsch J, Schoop R, Kuenzle U, Suter A. Echinacea alkylamides modulate TNF-alpha gene expression via cannabinoid receptor CB2 and multiple signal transduction pathways. Febs Lett. 2004;577(3):563-569.
- Mackie K. Cannabinoid receptors as therapeutic targets. Annu Rev Pharmacol Toxicol 2006;46(1):101.
- Sasagawa M, Cech NB, Gray DE, Elmer GW, Wenner CA. Echinacea alkylamides inhibit interleukin-2 production by Jurkat T cells. Int Immunopharmacol. 2006;6(7):1214-1221.
- Chen Y, Fu T, Tao T, et al. Macrophage activating effects of new alkamides from the roots of Echinacea species. J Nat Prod. 2005;68(5):773-776.
- Spelman K, Iiams-Hauser K, Cech NB, Taylor EW, Smirnoff N, Wenner CA. Role for PPARγ in IL-2 inhibition in T cells by Echinacea-derived undeca-2E-ene-8,10-diynoic acid isobutylamide. Int Immunopharmacol. 2009;9:1260-1264.
- Spelman K. The Pharmacodynamics, Pharmacokinetics and Clinical Use of Echinacea purpurea. America’s Pharmacist. 2012;Octobet 41-54.
- Spelman K. The extraction, stability, metabolism and bioactivity of the alkylamides in Echinacea spp. Exeter, U.K.: School of Biosciences, University of Exeter; 2009.
- Raduner S, Majewska A, Chen J-Z, et al. Alkylamides from Echinacea Are a New Class of Cannabinomimetics: Cannabinoid Type 2 Receptor-Dependent and -Independent Immunomodulatory Effects. J Biol Chem. 2006;281(20):14192-14206.
- Spelman K. Screening of Echinacea purpurea & E. angustifolia alkylamides for PPAR gamma activity. In: University of North Carolina 2009.
- Gulledge TV, Collette NM, Mackey E, et al. Mast cell degranulation and calcium influx are inhibited by an Echinacea purpurea extract and the alkylamide dodeca-2E,4E-dienoic acid isobutylamide. J Ethnopharmacol. 2018;212:166-174.
- Bauer R. [Echinacea drugs–effects and active ingredients]. Z Arztl Fortbild. 1996;90(2):111-115.
- Bauer R. Echinacea: Biological effects and active principles. In: Lawson LD, Bauer R, eds. Phytomedicines of Europe: Chemistry and Biological Activity. Washington, DC: American Chemical Society; 1998:140-157.
- Meeran MFN, Laham F, Azimullah S, et al. β-Caryophyllene, a natural bicyclic sesquiterpene attenuates β-adrenergic agonist-induced myocardial injury in a cannabinoid receptor-2 dependent and independent manner. Free Radic Biol Med. 2021;167:348-366.
- Dalby-Brown L, Barsett H, Landbo AKR, Meyer AS, Molgaard P. Synergistic Antioxidative Effects of Alkamides, Caffeic Acid Derivatives, and Polysaccharide Fractions from Echinacea purpurea on in Vitro Oxidation of Human Low-Density Lipoproteins. J Agric Food Chem. 2005;53(24):9413-9423.
- Thygesen L, Thulin J, Mortensen A, Skibsted LH, Molgaard P. Antioxidant activity of cichoric acid and alkamides from Echinacea purpurea, alone and in combination. Food Chemistry. 2007;101(1):74-81.
- Kashiwada Y, Takanaka K, Tsukada H, et al. Sesquiterpene glucosides from anti-leukotriene B4 release fraction of Taraxacum officinale. J Asian Nat Prod Res. 2001;3(3):191-197.
- Extract Database. International Center of Phytotherapy; 2006. Accessed July 18, 2006.
- Schutz K, Carle R, Schieber A. Taraxacum – A review on its phytochemical and pharmacological profile. J Ethnopharmacol. 2006;107(3):313-323.
- Hu C, Kitts DD. Dandelion (Taraxacum officinale) flower extract suppresses both reactive oxygen species and nitric oxide and prevents lipid oxidation in vitro. Phytomed. 2005;12(8):588-597.
- Bensky D, Gamble A, Kaptchuk TJ. Chinese herbal medicine : materia medica. Rev. ed. Seattle, Wash.: Eastland Press; 1992.
- Nadkarni KM, Nadkarni AK. Indian materia medica. 3d ed. Bombay,: Popular Book Depot; 1955.
- Blumenthal M, Busse WR, Bundesinstitut fèur Arzneimittel und Medizinprodukte (Germany). The complete German Commission E monographs, Therapeutic guide to herbal medicines. Austin, Texas: American Botanical Council; Integrative Medicine Communications; 1998.
- Smyth G. Practical Observations on the Therapeutic Effects of Taraxacum, With Cases. Lancet. 1845;46(1158):506-508.
- Clare B, Conroy R, Spelman K. The diuretic effect of a hydroethanolic extract of Taraxacum officinale folium on Human Subjects. Submitted for publication. 2007.
- Hawkins RG, Houston MC. Is Population-Wide Diuretic Use Directly Associated With the Incidence of End-Stage Renal Disease in the United States?: A Hypothesis. Am J Hypertens. 2005;18(6):744.
- Hu C, Kitts DD. Luteolin and luteolin-7-O-glucoside from dandelion flower suppress iNOS and COX-2 in RAW264.7 cells. Mol Cell Biochem. 2004;265(1-2):107-113.
- Hu C, Kitts DD. Antioxidant, prooxidant, and cytotoxic activities of solvent-fractionated dandelion (Taraxacum officinale) flower extracts in vitro. J Agric Food Chem. 2003;51(1):301-310.
- Sumanth M, Rana A. In vivo antioxidant activity of hydroalcoholic extract of Taraxacum officinale roots in rats. Indian J Pharmacol. 2006;38(1):pNA.
- Zhu M, Wong PY, Li RC. Effects of Taraxacum mongolicum on the bioavailability and disposition of ciprofloxacin in rats. J Pharm Sci. 1999;88(6):632-634.
- Yoshino K, Ogawa K, Miyase T, Sano M. Inhibitory effects of the C-2 epimeric isomers of tea catechins on mouse type IV allergy. J Agr Food Chem. 2004;52(15):4660-4663.
- Alvarez P, Alvarado C, Mathieu F, Jimenez L, De la Fuente M. Diet supplementation for 5 weeks with polyphenol-rich cereals improves several functions and the redox state of mouse leucocytes. Eur J Nutr. 2006;45(8):428-438.
- Minami K, Nakasugi T, Sun HD, et al. Isolation and identification of histamine-release inhibitors from Pistacia weinmannifolia J. Pisson ex. Franch. J Natural Med. 2006;60(2):138-140.
- Jackob Moskovitz MBYaPBC. Free Radicals and Disease. Arch Biochem Biophys. 2002;397(2):354.
- Ding S, Jiang H, Fang J. Regulation of Immune Function by Polyphenols. J Immunol Res. 2018;2018:1264074.
- Cortes JR, Perez-G M, Rivas MD, Zamorano J. Kaempferol Inhibits IL-4-Induced STAT6 Activation by Specifically Targeting JAK3. Unidad de Investigacion, Hospital San Pedro de Alcantara, Caceres, Spain. Journal of Immunology (2007), 179(6), 3881-3887. Publisher: American A. J Immunol. 2007;179(6):3881-3887.
- Guzik TJ, Korbut R, Adamek-Guzik T. Nitric oxide and superoxide in inflammation and immune regulation. 2003;54(4):469-487.
- Kim HM, Lee EH, Shin TY, Lee KN, Lee JS. Taraxacum officinale restores inhibition of nitric oxide production by cadmium in mouse peritoneal macrophages. Immunopharmacol Immunotoxicol. 1998;20(2):283-297.
- Hudec J, Burdova M, Kobida L, et al. Antioxidant Capacity Changes and Phenolic Profile of Echinacea purpurea, Nettle (Urtica dioica L.), and Dandelion (Taraxacum officinale) after Application of Polyamine and Phenolic Biosynthesis Regulators. J Agric Food Chem. 2007;55(14):5689-5696.