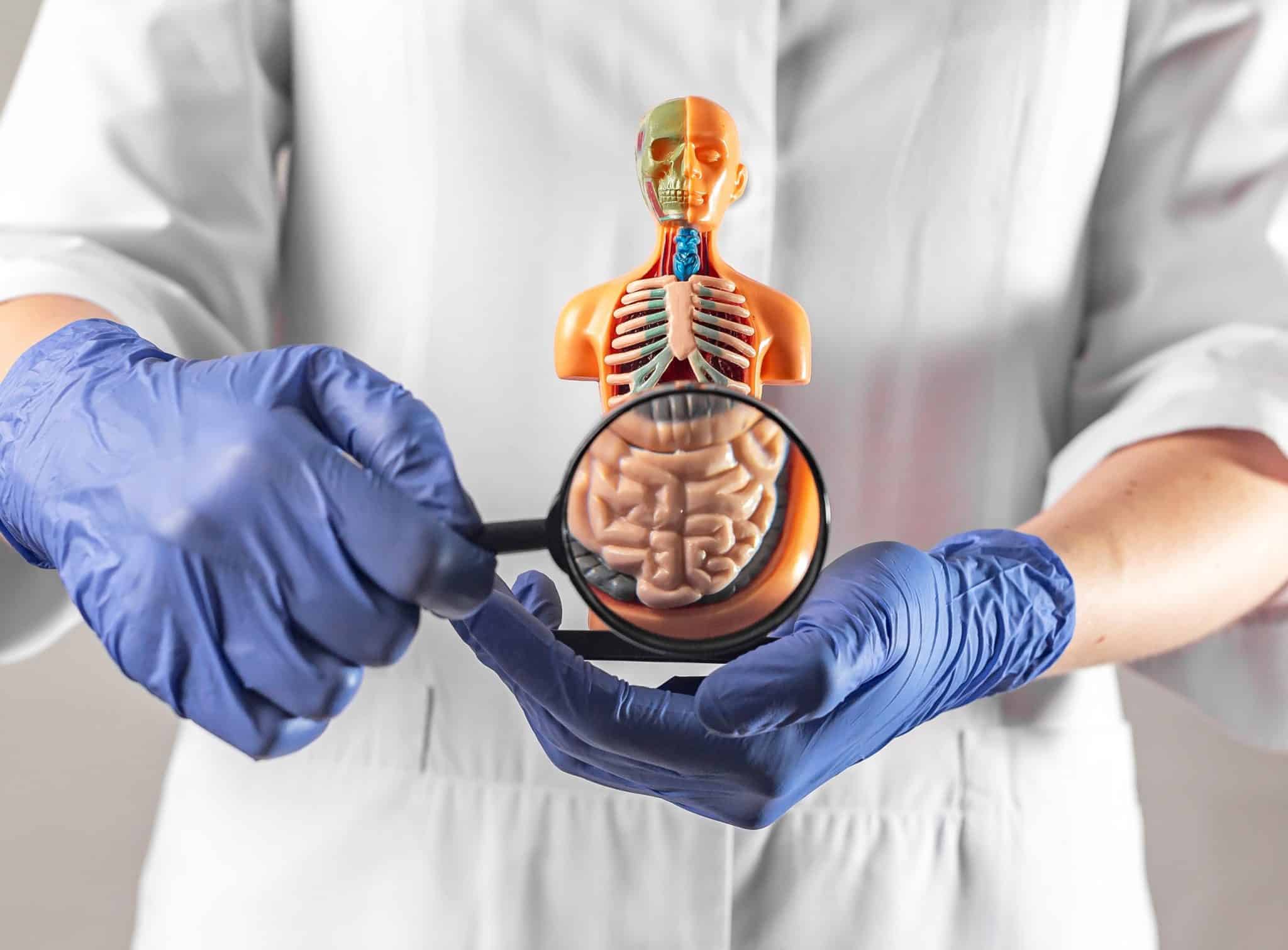
In this major review we will consider the astonishing implications of discoveries that each of us is a unique joint enterprise between our body and its resident microbes. No longer are our commensal bacteria and viruses seen as add-ons. Instead, we now know there is a dynamic relationship between our microbiota and the rest of us which really does determine who and how healthy we are.
Microbial communities inhabit all body surfaces and cavities. However, the microbiota in the gut are by far the most substantial and have the greatest impact on our lives and health.
We will reflect on how the therapeutic journey in supporting the gut microbiome has moved from probiotics, through prebiotics and now to consider the profoundly intriguing notion that plants could be ‘postbiotics’, reflecting the way food materials and their metabolites engage in ‘crosstalk’ between our microbiota and the rest of us.
We will see that plant constituents may indeed have the biggest dietary impact on the health of the gut microbiome, and that a leading contribution to our overall health may yet turn out to be their role in facilitating a healthy collaboration between us and our ‘inner garden’.
There are also implications for bioavailability and dosage. Plant constituents are most likely to be active in the gut when they are poorly absorbed, and even then, given the impact being expected, only a few will deliver benefits in less than dietary doses. We will look at the range of plant influences on the microbiome with these practicalities in mind.
The gut microbiome: Our inner garden
What is the microbiome?
The gastrointestinal tract harbours a diverse microbial community of which up to 99.99% are anaerobic bacteria. Concentrations as high as 1011/ml may be found in the colon, with densities thinning upstream, towards near sterility in the stomach. The bacteria are classified into four main phyla and up to 1000 distinct species, with the most dominant, Faecalibacterium prausnitzii making up much of the stool bulk. Viruses, archaea and eukaryotes (such as Candida) are also normally present at varying levels.
Phylum | Summary | Commonly detected constituent genera |
---|---|---|
Actinobacteria | Gram positive | Atopobium, Bifidobacterium(I), Collinsella, Eggerthella |
Bacteroidetes | Gram negative bacilli Typically obligately anaerobic | Alistipes, Bacteroides(III), Barnesiella, Parabacteroides, Prevotella |
Firmicuttes | Gram positive Uusually abundant and dominant members of the gut microbiota Contains the greatest degree of species diversity Dominant phylum of bacteria closest to the gut wall | Clostridia class (obligate anaerobes) Acidaminococcus, Anaerostipes, Anaerotruncus, Blautia, Butyrivibrio, Clostridium(II), Coprobacillus, Coprococcus, Dialister, Dorea, Faecalibacterium(I), Finegoldia, Lachnospira(I), Megasphaera, Phascolarctobacterium, Roseburia, Ruminococcus(II), Subdoligranulum, Veillonella Bacilli class (typically facultative anaerobes) Enterococcus(II), Lactobacillus(I), Streptococcus(II) |
Proteobacteria | Gram negative Typically facultatively anaerobic species Includes many conventional and opportunistic pathogenic species | Alcaligenes, Bilophila, Campylobacter(II), Desulfovibrio(II), Enterobacter(III), Escherichia(III), Hafnia, Helicobacter(II), Klebsiella(II), Oxalobacter, Parasutterella, Proteus(III), Sutterella |
Other phyla | Only a limited number of species | Fusobacterium, Akkermansia(I), Victivallis |
It is important to note that the microbiome population is stratified. There is a resilient commensal population, mostly species of the Firmicutes phylum, that are adherent to the gut wall. These residents provide the most consistent element in the community, readily reclaim their niche when disturbed and compete against interlopers. Further away from the gut wall are more transient populations, largely evacuated with stool movements.
Each person’s microbiome is unique – and always changing (to some extent with each meal). Even identical twins share only 50% of their microbiome profile (1). However, healthy microbiomes are resilient, able to return to steady state after being perturbed (2).
What does the microbiome do?
Overall the microbiome contains at least 100 times as many genes as the human genome. Not surprisingly, therefore, the microbiota mediate many functions, including modulating gene expression in the host. There is no function in the body that is not affected, with digestion, metabolism, detoxication, circulation, and hormonal, nervous, immune and inflammatory activities notably influenced. For example, beneficial microbiota induce expression of opioid and cannabinoid receptors (comparably to morphine) (3), modulate inflammatory cytokines, reduce obesity and symptoms of anxiety and depression (4).
People with Alzheimer’s disease, Parkinson’s, depression and irritable bowel syndrome have been shown to have significant differences in gut bacteria when compared to healthy people (2). There is particular consensus that changes in the microbiome are critical for metabolic syndrome conditions like insulin resistance, non-alcoholic fatty liver disease (NAFLD), obesity and diabetes (5,6). Intriguingly, it may even be that one of the most widely used medicines for diabetes may mediate its benefits partly through changes in the microbiome (7)! There is also an emerging concept of the ‘microbiome-gut-brain axis’ to augment the widely understood ‘gut-brain axis’ with the important role of microbial metabolites on brain function (8).
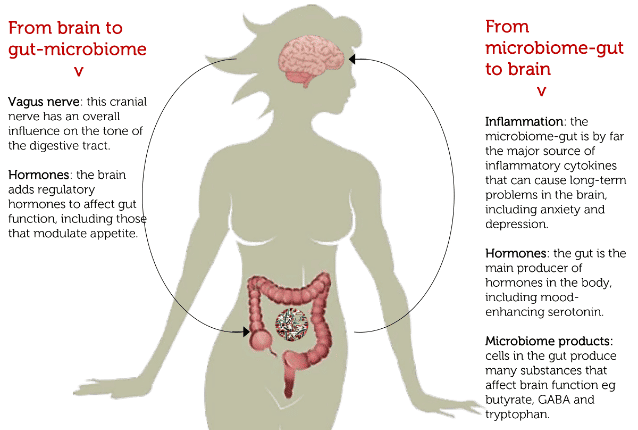
Fortunately innate microbiome resilience and a diversity of phenotypes ensures core ‘housekeeping’ functions are maintained (9). These include:
Metabolism and nutrition:
- vitamin synthesis (e.g. vitamin K and folate);
- regulating the uptake and deposition of dietary lipids;
- converting otherwise indigestible dietary elements, e.g. lactose (into glucose and fructose) and alcohols (into short-chain fatty acids: SCFAs).
Protection:
- maintenance of intestinal barrier integrity;
- barricading against invading pathogens by competitive exclusion (production of antimicrobial peptides e.g. non-specific fatty acids and peroxides, specific bacteriocins);
- engagement of attachment sites;
- reduction in host stress responses (microbiota–gut–brain axis).
Immunomodulation:
- inducing tolerance to dietary and microbial antigens;
- activating intestinal dendritic cells;
- induction of IgA from B cells.
How to manage a biome?
Our gut microbiome shares many of the properties of other complex self-organizing ecological systems of mutually interacting living organisms. An obvious parallel is the soil biome that nourishes the plants that grow in it, and which for example is the basis of new insights into the ‘wood-wide web’ through which trees are known to communicate with each other.
A biome provides an immediate challenge to the usual reductionist approach to biological and medical science. In the case of the gut microbiome this means:
- it can never be fully measured as it is in constant flux: any culture or -omic reading will be partial and soon out of date;
- it cannot be controlled, as the interactions between its thousands of elements are unknowable: even faecal implants need to bed-in with resident flora and to find a new modus vivendi.
A quick insight is that managing the inner microbiome requires a respect comparable to that in managing a healthy soil, as is the aim for example of the organic farmer. It requires nurturing rather than manipulation.
Above all is the recognition that this biome is in communication with the rest of us – it engages in ‘crosstalk’. We will come back to this in the next section.
Current gut microbiome management approaches
There are two familiar therapeutic approaches to maintaining a healthy microbiome, the probiotic and prebiotic. We argue here for the inclusion of a third emerging term ‘postbiotic’ to apply to a wide range of plant constituent effects.
Probiotics are living organisms added to the gut in the hope that they may seed new colonies. They’re usually added to yoghurts, provided as fermented foods or taken as food supplements. There is evidence that probiotics may be helpful in some cases, such as helping prevent diarrhoea when taking antibiotics (10), reducing symptoms of lactose intolerance (11), and helping to ease some symptoms of irritable bowel syndrome (IBS)(12), but for example there is no clear benefits in reducing diarrhoea generally (13), and other evidence for benefits remains scanty.
The clear challenge with introducing live organisms orally is that a healthy stomach is a sterilising chamber (14). This suggests more benefits might arise with low stomach acid conditions (possibly marked by a tendency to gut infections), including those induced on purpose by regular use of antacids and PPH drugs like omeprazole. Even in a healthy stomach a tiny percentage of live organisms may survive and could quickly build populations further down the gut; however, they then still face competition from the resident flora.
Prebiotics are generally defined as plant materials that can support a healthy gut microbiome. They include the inulin-type fructans, which are linked by beta (2-1) bonds that limit their digestion by upper intestinal enzymes, and fructo-oligosaccharides. Both can reduce levels of various Firmicutes species and increase those of Bacteroidetes, as well as improving glucose sensitivity, fat metabolism, and inflammatory responses. Specifically, beta-glucan induces the growth of Prevotella and Roseburia with a concomitant increase in SCFA propionate production (15). Inulin and all oligosaccharides have a strong bifidogenic effect. A high fructo-oligosaccharide and fibre diet has led to significant increases in butyrate-producing Bifidobacterium (16). These compounds are present in significant amounts in many edible fruits and vegetables, including wheat, onion, garlic, leeks, artichokes, and bananas.
Fibre, including resistant starch and non-starch polysaccharide dietary components such as cellulose, also exerts prebiotic effects (17). Many of the postulated benefits of consuming a diet that is high in fibre may be mediated, at least in part, via short-chain fatty acid (SFCA) production by intestinal microbes. As well as nourishing the gut wall tissues, SCFAs have anti-inflammatory properties (18) (see also in the next section).
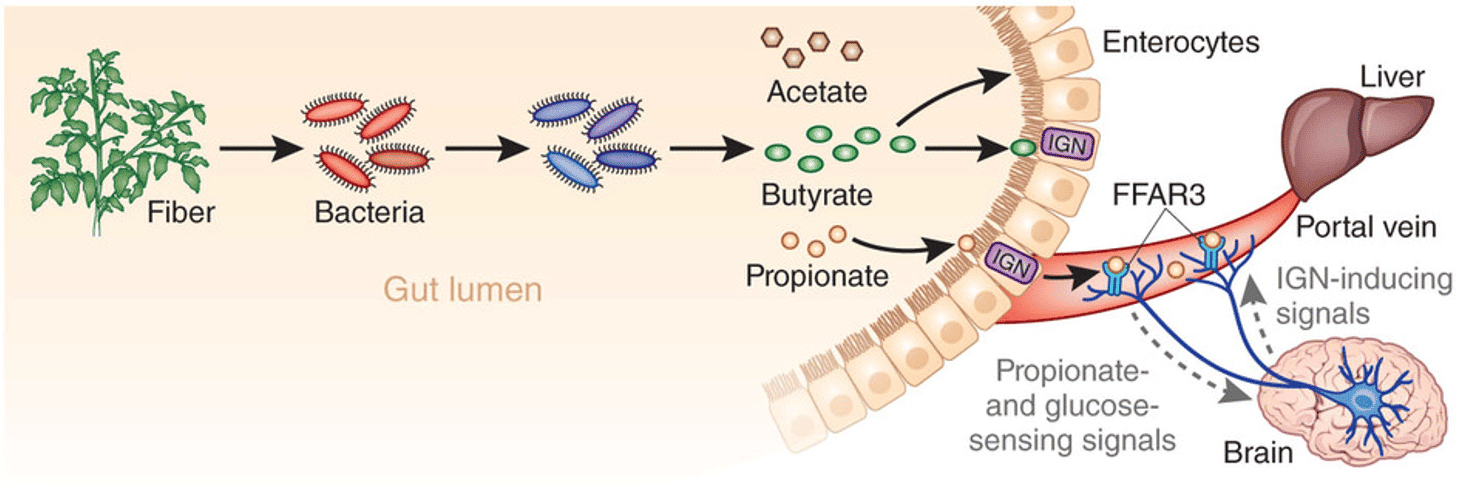
It is known that changes in diet can affect SCFA profiles. For example, obese individuals fed low-carbohydrate diets showed severe depletion in faecal butyrate levels, probably as a result of reduced abundance of butyrate-producing gram-positive Firmicutes species following the change in diet. Similarly, European children have lower amounts of SCFAs in their faeces than high-fibre consuming rural African children. Moreover, fibre also acts to generate faecal bulk, decrease transit time, and therefore reduce exposure of the intestinal lumen to harmful or toxic compounds (19). Increasing the range of carbohydrate substrates for microbial metabolism to SCFA’s is increasingly seen as critical for good metabolic health and to protect against metabolic problems like obesity and diabetes (20).
As we will see, there are many other plant constituents that are being shown to have prebiotic effects. However, these benefits most often come secondarily out of bacterial transformation in the gut.
Cross talk: Mutual regulation
It’s a team effort
Our inner biomes are not independent universes. A healthy gut microbiome is a product of a dynamic equilibrium with its host. There are extensive interactions between our microbiome and the rest of us and this conversation could be the main factor in maintaining the health of both. It certainly opens many new prospects for therapeutic interventions.
There are many mechanisms already identified by which the host and microbiota can influence each other.
1. Host secretions and receptors
Mucins: Goblet cells in the gut lining secrete these glycoproteins to form mucous layers as an innate frontline defence mechanism. Near the gut wall are several adherent layers that are also home to the most resilient bacteria. Further out there are less adherent mucin layers which are eliminated by peristaltic movement. There is evidence that mucin production is also a protective response in inflammatory events (21).
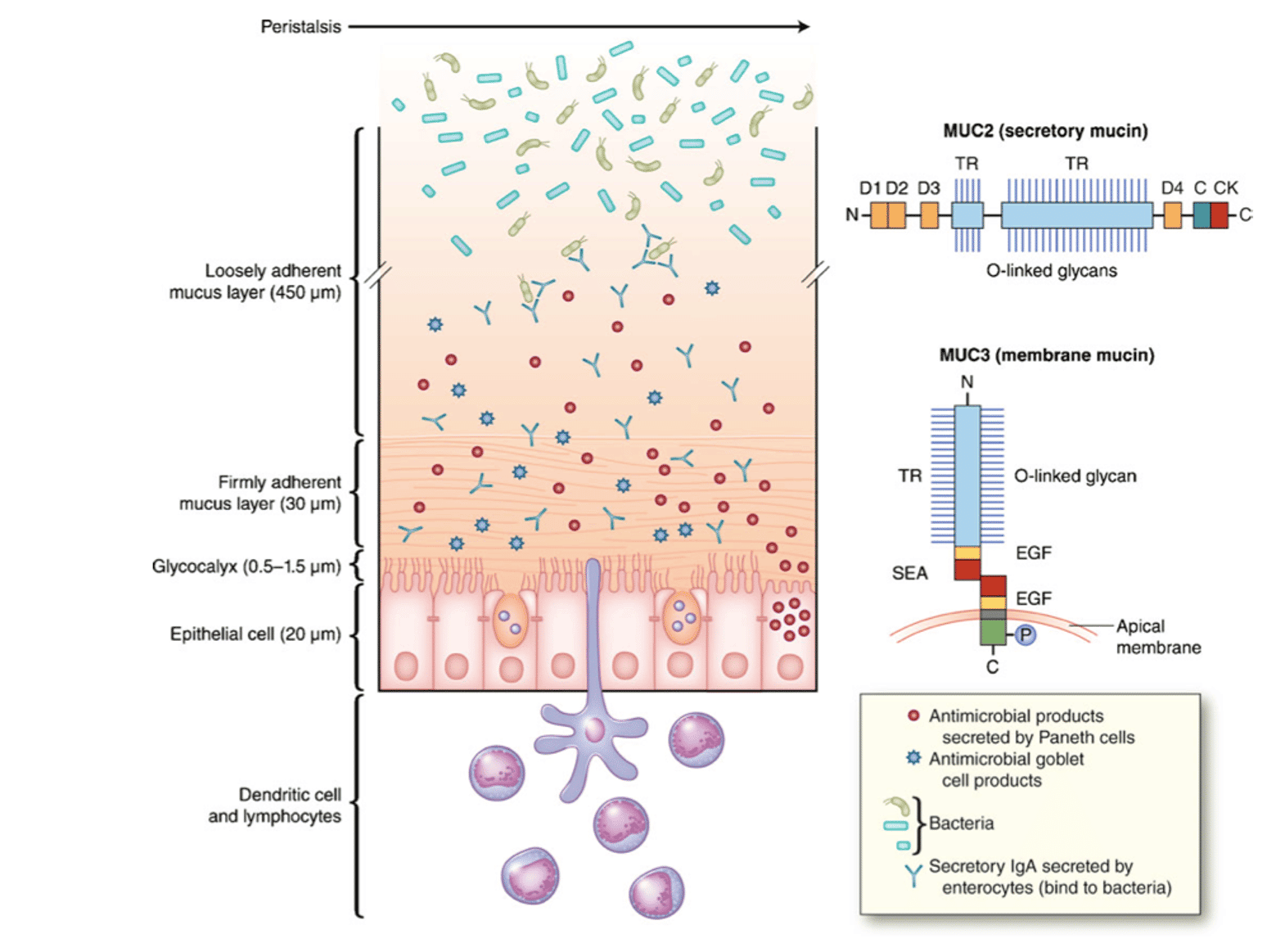
Enteroendocrine cells: These are the primary chemoreceptors and modulators of appetite and digestion in the GI lumen. They appear to be sophisticated “tastebuds” responding to changes luminal content, especially those mediated by bacteria, by releasing chemical messengers such as cholecystokinin, ghrelin and secretin that in turn activate neuronal pathways. They could also be microbiota-operated gateways to the gut-brain axis (22). Among other functions, they express glucagon-like peptide-1 (GLP-1), that plays a key role in metabolic health and protection against diabetes. Among other ligands are curcuminoids from turmeric (23).
Enteroendocrine cells appear to have two distinct sensing pathways, to nutrients and bacteria. The former are known to affect enteroendocrine function after absorption (i.e. systemically) so may reach these cells from behind. On the opposite side the cells have a small narrow opening to the luminal surface and it is possible this may sense bacterial inputs. Some bacterial Toll-like receptors (e.g. TLRs 4, 5, and 9) are exclusively expressed on enteroendocrine cells. When these are stimulated by bacterial ligands (e.g. LPS or flagellin) CCK and other chemokines are secreted (24).
Enterochromaffin cells: These are the body’s most abundant endocrine cells and produce about 90% of the body’s serotonin (5-HT). They serve as bidirectional transducers that modulate the relationship between the gastrointestinal lumen and the nervous system, sensing the luminal content of the gut and controlling the function of the whole digestive tract. Microbial influence on enterochromaffin cells suggest a role in the regulation of visceral pain. Volatile components of herbs and spices are ligands for olfactory receptors on enterochromaffin cells, now seen as a relatively accessible target for the treatment of IBS, of conditions following anticancer therapy, and other gastrointestinal disorders (25).
Bitter receptors: Once thought to be restricted to the mouth, there are up to 30 types of G-protein coupled bitter taste receptors (T2Rs) throughout the body, activated by numerous ‘tastants’. T2Rs in the bowel (e.g. T2R38) are sensitive to bacterial metabolites. T2Rs have been thought to serve as ‘danger receptors’, to respond to potentially harmful substances, and are upregulated by inflammation. There is also evidence that T2Rs in the gut wall are co-located with enteroendocrine cells, marked by chromogranin A and a-gustducin reactivity. The latter is associated with expression of cholecystokinin, GLP-1, PYY and ghrelin from enteroendocrine cells. These in turn modulate appetite, increase fat metabolism and insulin sensitivity (26). The herbal materia medica has many bitter principles that are likely to be active on these receptors.
Olfactory receptors: Exciting research has identified chemosensitivity in cells around the body mediated by the same sensors that mediate taste and smell. They have been identified in the airways, throughout the gut lining, kidneys, the microvasculature, brain, muscles and pancreas. A key olfactory G protein-coupled receptor, initially discovered in the renal juxtaglomerular apparatus of the kidney is Olfr78, also found throughout the vasculature. Among Olfr78’s ligands are propionate (the microbiome that generates this SCFA has also been shown to alter blood pressure) (27).
2. Microbiome products
The microbiome can generate a wide range of metabolites with activity on host functions. Many of importance will feature when we consider their impact on bile acids, and on various types of plant constituents below. However their greatest single impact on host functions is their generation of short chain fatty acids.
SCFAs: A universal activity of the intestinal microbiota is the breakdown and fermentation of carbohydrates into short chain fatty acids, principally acetate, propionate and butyrate. SCFAs are rapidly taken up by the colonic mucosa, contributing up to 10% of the body’s calorific needs, and act as important stimuli for the absorption of sodium and water by the colon. Intestinal epithelial cells derive as much as 60–70% of their energy needs from butyrate and this SCFA has important effects on intestinal barrier integrity and repair and mucin production. All SCFAs reduce intestinal inflammation, although there is less robust evidence for benefits on metabolic health (28).
Bacterial metabolites also include non-SCFAs like amino acid metabolites and bacterial amyloids also with protective effects on host health (29).
Bile: The gut coach?
Bile is a major modulator of gut performance (in former times it was referred to as ‘endogenous senna’). Increasing bile production and elimination (with choleretics and cholagogues respectively) has been a productive focus among herbal practitioners in correcting bowel and other digestive problems. Bile also has a major role in managing the microbiome, with overgrowth a complication of cholestasis and liver disease. Bile and the microbiome have an extremely complex interaction, with bacteria processing bile acids (BAs) to a variety of more or less irritating or even toxic metabolites, potentially a key factor in inflammatory bowel diseases, hepatitis and bowel cancer (30).
Apart from their detergent properties in lipid digestion, BAs have been recognized to control a range of metabolic and immune functions throughout the body. These effects are mediated via dedicated receptors. One is farnesoid X receptor (FXR). In the gut this maintains epithelial barrier integrity, including by induction of healthy microbe populations. Overall the microbiome induces FXR-dependent suppression of both BA synthesis in the liver and BA import from the ileum. The FXR receptor not only affects cholesterol metabolism but is also involved in the body’s sugar and fat metabolism (31).
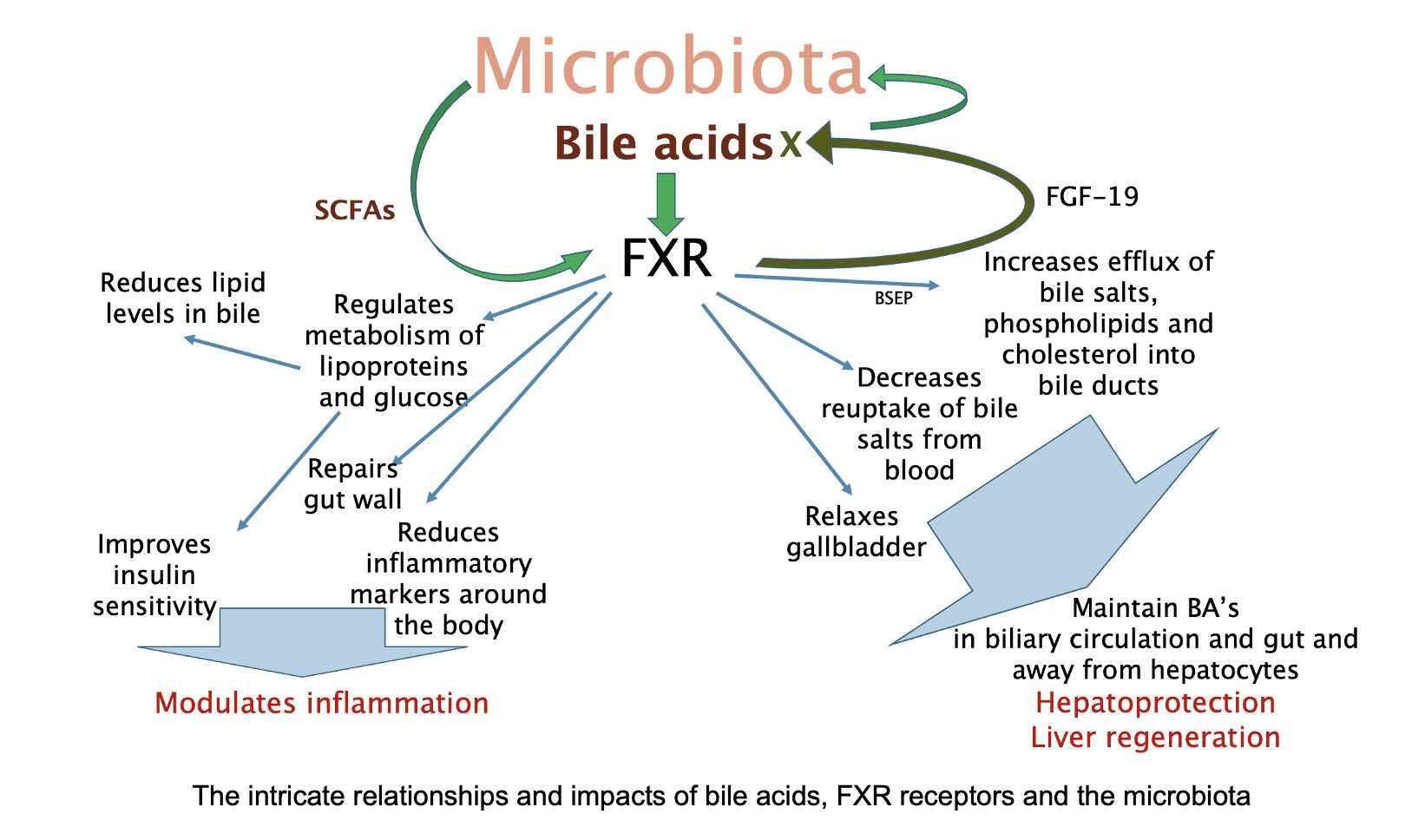
Postbiotics: Crosstalk in action?
Postbiotics have been defined as soluble factors secreted by live bacteria, or released after bacterial lysis, which may have anti-inflammatory, immunomodulatory, anti-obesogenic, antihypertensive, hypocholesterolemic, anti-proliferative, and antioxidant activities, and that may contribute to the improvement of host health (32).
The term has come to mean different things in various contexts and a consensus definition is still elusive. For example ‘postbiotic supplements’ are industrially produced by bacterial vat fermentation, to complement probiotics. Locking the term as product related, the International Scientific Association for Probiotics and Prebiotics (ISAPP) in 2021 defined a postbiotic as “a preparation of inanimate microorganisms and/or their components that confers a health benefit on the host” (33). A variation ‘parabiotic’ refers to denatured bacterial material that is used instead of probiotics. However other medical sources without a product focus equate ‘postbiotics’ with short chain fatty acids (SCFAs), and the authoritative Harvard Health for example adds nutrients such as vitamins B and K, amino acids, as well as antimicrobial peptides that slow the growth of harmful bacteria (34).
This latter direction suggests the use of the term ‘postbiotic’ for any product of bacterial metabolism in the gut. This would conveniently fill a gap in the range of dietary benefits for the microbiome, as the term ‘prebiotic’ is consistently restricted to fibre and dietary carbohydrates referred to above (35). The many examples of other plant constituents with beneficial effects on gut microbiota increasingly are known to rely on microbial metabolism, and may even engage in host-microbe crosstalk. Some of these products are likely to generate ‘postbiotic’ effects, both on the microbiome and on host health. There is an early review of these issues in relation to Chinese herbs (36). We will look at some examples in the following section.
Plants and the microbiome
All plant foods support a healthy gut biome
It appears that a plant-based diet generally promotes the development of a more diverse microbiome. Vegetarian/vegan diets are associated with higher levels of protective biota such as Prevotella and Ruminococcus along with lower levels of bile-consuming Bacteroides and generally other genera of the Firmicutes phylum (37,38).
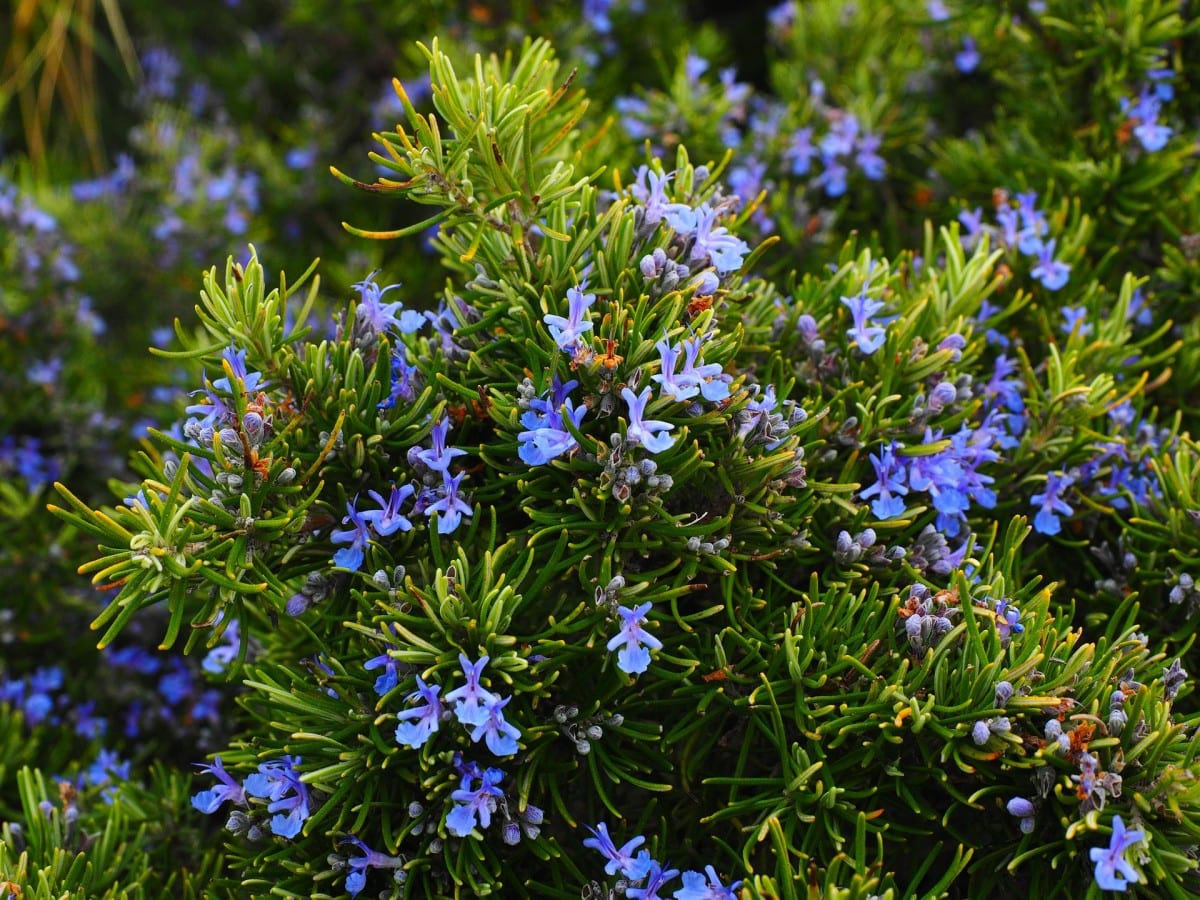
Most spices and kitchen herbs have been shown to promote the growth of Bifidobacterium spp. and Lactobacillus spp. to varying degrees. A combination of spices exhibited high inhibitory activity against Ruminococcus species, though minimal activity against selected strains of Bacteroides, Finegoldia, E. coli, Salmonella, and Staphylococcus. Fusobacterium spp. were susceptible to cinnamon, and F. necrophorum also to oregano and rosemary. Cinnamon exhibited modest activity against toxigenic C. difficile and cinnamon, rosemary, or turmeric against a few strains of other Clostridium. Oregano, black pepper, and ginger possessed prebiotic‐like effects by promoting the growth of beneficial bacteria in one hand and suppressing pathogenic bacteria on the other (39).
There is interesting evidence of herbal effects on the microbiome from researchers in traditional Chinese medicines (40,41). One recent line of enquiry here is that the presence of polysaccharides in some traditional herbs may optimise bioavailability of active constituents, perhaps through an interaction with the microbiota (42).
However much of the evidence so far relates to particular plant constituents. We have already seen the role of plant carbohydrate and fibre as prebiotics. What is emerging is that many other elements engage with the microbiota. As is often the case it is helpful in assessing plant and herbal impacts to revert to the archetypal constituents that would have been recognised in traditional pharmaceutics, by appearance, taste and smell and other immediate impacts after consumption. In the sections below these groups also stand out because they all have their own immediate impact on gut physiology, apart from any on the microbiome.
Mucilages and gums
The ‘slimy’ quality of some plants provides well-known soothing and healing properties externally and internally, based on the physical properties of these complex carbohydrates. It appears that at least one example, gum arabic, performs even better as a prebiotic than inulin. In one study, 10g daily of gum arabic consumed by healthy volunteers led to significantly higher numbers of gut Bifidobacteria, Lactobacilli and Bacteroides than inulin controls (43). In another controlled clinical trial the reduction in inflammatory markers and symptom relief in rheumatoid arthritic patients after 30g daily of gum Arabic was considered to follow microbiomic engagement (44).
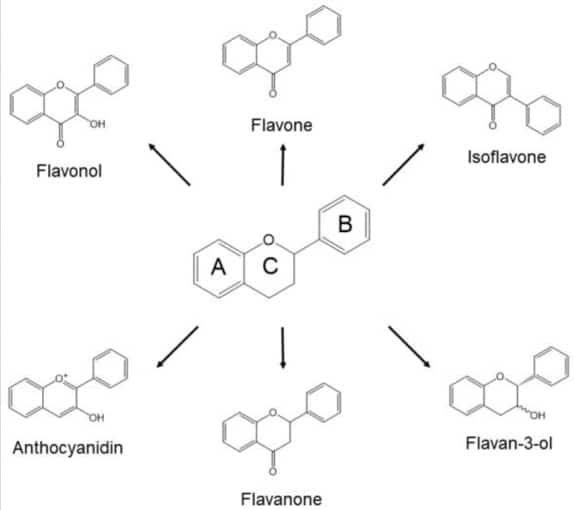
Polyphenols
These ubiquitous molecules, some long recognised by our ancestors for their colouring in plants, and linked to many traditional medicinal uses such as fever and inflammation management, have presented a therapeutic challenge.
In spite of a huge and growing literature on their benefits in the diet and medicine, their bioavailability is notably limited.
A first breakthrough was the realisation that the relatively cumbersome polyphenolic compounds are metabolised into much more accessible and active simple phenols – by the gut bacteria.
The fundamental role of the gut microbiota in realising the health benefits of these important phytonutrients immediately suggests that we should consider polyphenols as interacting with these organisms, even engaging in the crosstalk between host and gut biome, and that any use of the term ‘prebiotic’ becomes insufficient to characterise this relationship. The polyphenol metabolites produced by gut bacteria do meet at least one definition of ‘postbiotic’ and we will offer this term for relevant plant constituents to follow.
Polyphenols generally interact with intestinal microbiota to activate SCFA production, intestinal immune function, and other protective physiological processes (45,46).
As one author noted: “This interplay provides the central explanation as to the stupefying pleiotropic biological/clinical actions and functions of “parent” polyphenols even though their systemic bioavailability is incredibly limited” (47).
In one research report, phenolic extracts obtained from eight berries containing anthocyanins, flavan-3-ols, B-type proanthocyanidins, and ellagitannins inhibited the growth of food poisoning bacteria, such as Salmonella, Listeria and Staphylococcus aureus, but did not affect the growth of the probiotic bacterium, Lactobacillus rhamnosus (48).
Any benefits of polyphenols on the microbiome would add to the case for the consumption of plants grown organically: a meta-analysis has shown a 20–60% increase in levels of polyphenols: flavonoids (eg flavanones, flavones, flavonols, anthocyanidins, isoflavones, and proanthocyanidins), and non-flavonoid polyphenols such as lignans (49). This is not surprising: polyphenols are generated by the plant in defence against grazing, infestation, infection and disease. Without agricultural pesticides and other external protections it could be expected that organically-grown plants would need more of their own resources (50).
We shall now look at specific plants whose effects on the microbiome appear to be mediated in part by polyphenols.
Cocoa
Cocoa polyphenols interact bidirectionally with the gut microbiota. They modulate the composition, enhancing beneficial gut bacteria, such as Lactobacillus and Bifidobacterium, and reducing the number of pathogens such as Clostridium perfringens. Microbial cocoa metabolites are themselves bioactive: they enhance gut health, display anti-inflammatory activities, positively affect immunity, and reduce the risk of various diseases (51).
Tea
Polyphenols from Camellia sinensis are metabolized by the microbiome and in turn promote the growth of beneficial species. They promote their growth in lean people and correct the dysbiosis found in obese people, correcting dysbiosis associated with high-fat diets and influencing the growth of some bacterial species involved in lipid or bile salts metabolism (52). Tea polyphenols are also able to hinder the growth of some pathogenic bacteria, especially gram-positive species; in general green tea reduces the production of pro-inflammatory substances such as lipopolysaccharides, and its compounds have been shown to correct the microbial dysbiosis associated with inflammatory diseases (53).
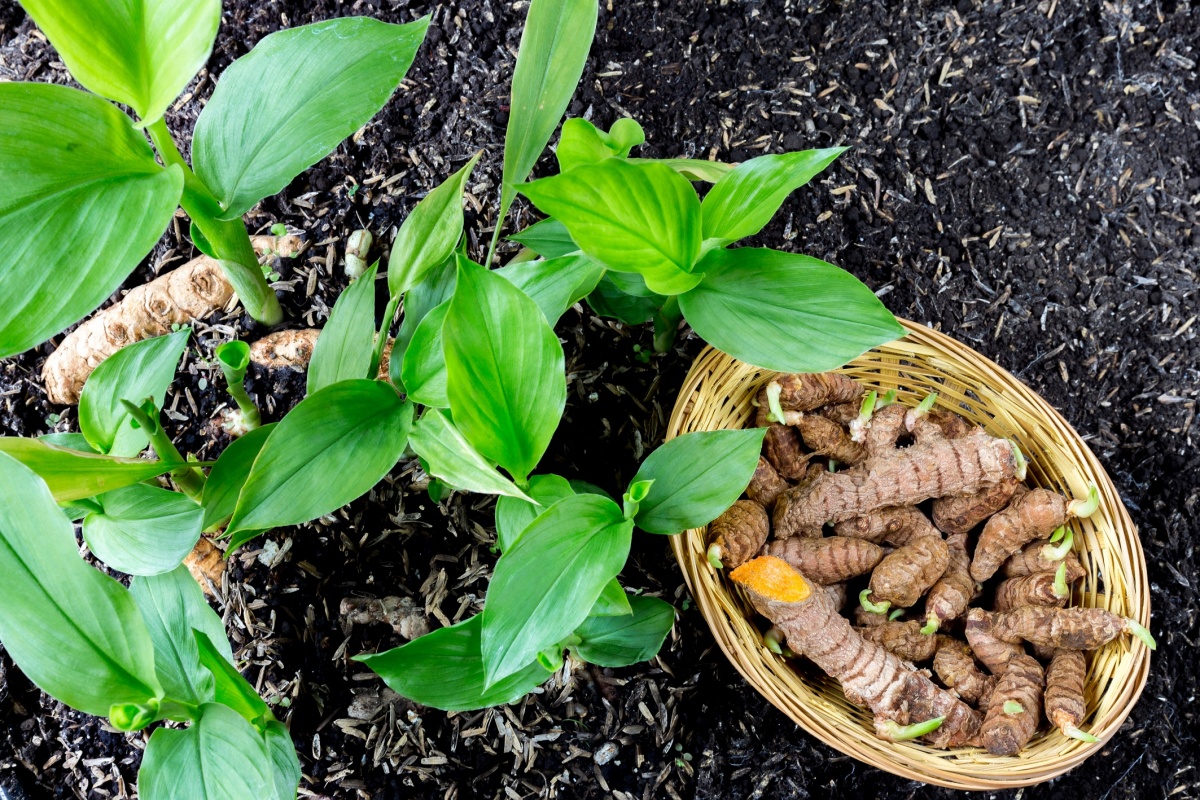
Turmeric
This spice is often referred to as a ‘prebiotic’. It increases butyrate-producing bacteria and faecal butyrate levels, and especially anti-inflammatory Bifidobacteria and Lactobacilli, and reduces proinflammatory Enterobacteria, Enterococci and Coriobacterales (54,55). Breath hydrogen increases when turmeric is added to meal indicating increased colonic carbohydrate metabolism (56).
However turmeric’s key polyphenol constituent curcumin cannot serve as a direct energy source for commensal microbiota and thus does not fully meet the definition of ‘prebiotic’. Instead its supportive effects on the microbiome are likely to be driven largely by indirect effects based on alterations in host physiology, which may include changes in barrier function or through selective survival of local bacteria or other microorganisms. Curcumin stimulates glucagon-like peptide-1(GLP-1) production from enteroendocrine cells (with potential benefits in reducing diabetes) (57); it also inhibits Toll-like receptors (TLR2, TLR4 and TLR9) to induce a cascade of signalling events that leads to proinflammatory cytokine production and is responsible for innate immune system activation. TLR4 in particular responds to bacterial lipopolysaccharides (58). Increasing the absorption of curcumin in the small intestine may reduce these potential benefits in the colon (59).
Curcumin is transformed by microbiota into more active metabolites (demethylcurcumin, bisdemethylcurcumin, dihydrocurcumin, tetrahydrocurcumin and ferulic acid) which have greater bioactivity, bioavailability and therapeutic potential than their parent. They increase microbiomic diversity, reduce absorption of inflammatory bacterial lipopolysaccharides (LPS) with quantifiable benefits for IBD, hepatic stenosis, tumorogenesis and neurological disease (60).
Turmeric further stimulates gallbladder emptying – by between 25-50% (61). By increasing microbiotic metabolism of secondary bile acid levels it can however reduce consequent laxative action (62). It was found bisacurone B was the most potent choleretic ingredient, followed by ar-turmerone, bisdemethoxycurcumin, demethoxycurcumin, and then curcumin (63).
Baical skullcap
Baicalin is the active polyphenolic constituent of this widely used medicinal herb, originating in China. This is involved in the interactions of the liver-gut axis by regulating TGR5, FXR, bile acids and the microbiota. It also regulates intestinal flora and gut wall health by promoting the production of SCFA-producing microbes such as Butyricimonas spp., Roseburia spp., Subdoligranulum spp., and Eubacterium spp (64). It also appears to regulate bile acid metabolism by increasing the production of bile salt hydrolase of bacterial origin (65).
Tannins
These astringent principles widely used in human history for their wound-healing are complex polyphenols that rely on the gut bacteria for much of their activity. Hydrolysable tannins, such as gallotannins and ellagitannins, are metabolised by the microbiome to generate gallic acid, ellagic acid, and their catabolites (66).
The formation of bioavailable human gut microbiota metabolites urolithin A, B and C has been established for elagitannin-rich aqueous extracts from the meadowsweet (Filipendula ulmaria), the oak (Quercus robur), Geum urbanum, and species of Geranium, Potentilla and Rubus. Significant inhibition of TNF-α production was determined for all urolithins, while for the most potent urolithin A, inhibition was observed at nanomolar concentrations. Urolithin C also inhibited IL-6 production (67).
Acrids
The heating remedies in traditional medicine, sourced in northern climes from sulfated constituents of the mustard and the onion families, and globally from the spices of Asia, have often been seen as the leading principles in defence against diseases. Given their concentrated activity doses can be relatively low and it is therefore likely that their systemic effects rely on reflex responses from stimulation of nerve fibres (pain or C-fibres) in the mouth and upper digestive tract. Nevertheless, it appears that these benefits do reach the gut microbiome.
Chilli
A short-term and high-dose capsaicin-enriched diet (10 mg/day, for two weeks) has been proven to increase beneficial Faecalibacterium prausnitzii levels in healthy humans; these organisms are inversely associated with IBD, immunity, obesity, diabetes, asthma, major depressive disorder, and colorectal cancer. Both low and high concentrations of dietary capsaicin (respectively 5 and 10 mg/day) administered for each one for 2 weeks in healthy subjects led to an increase in the ratio of the overall intestinal flora balance (68). Capsaicin and other constituents of chilli exert multiple benefits on gut microbiota and crosstalk, involving various and complex mechanisms, with benefits in mainly metabolic and inflammatory diseases (69).
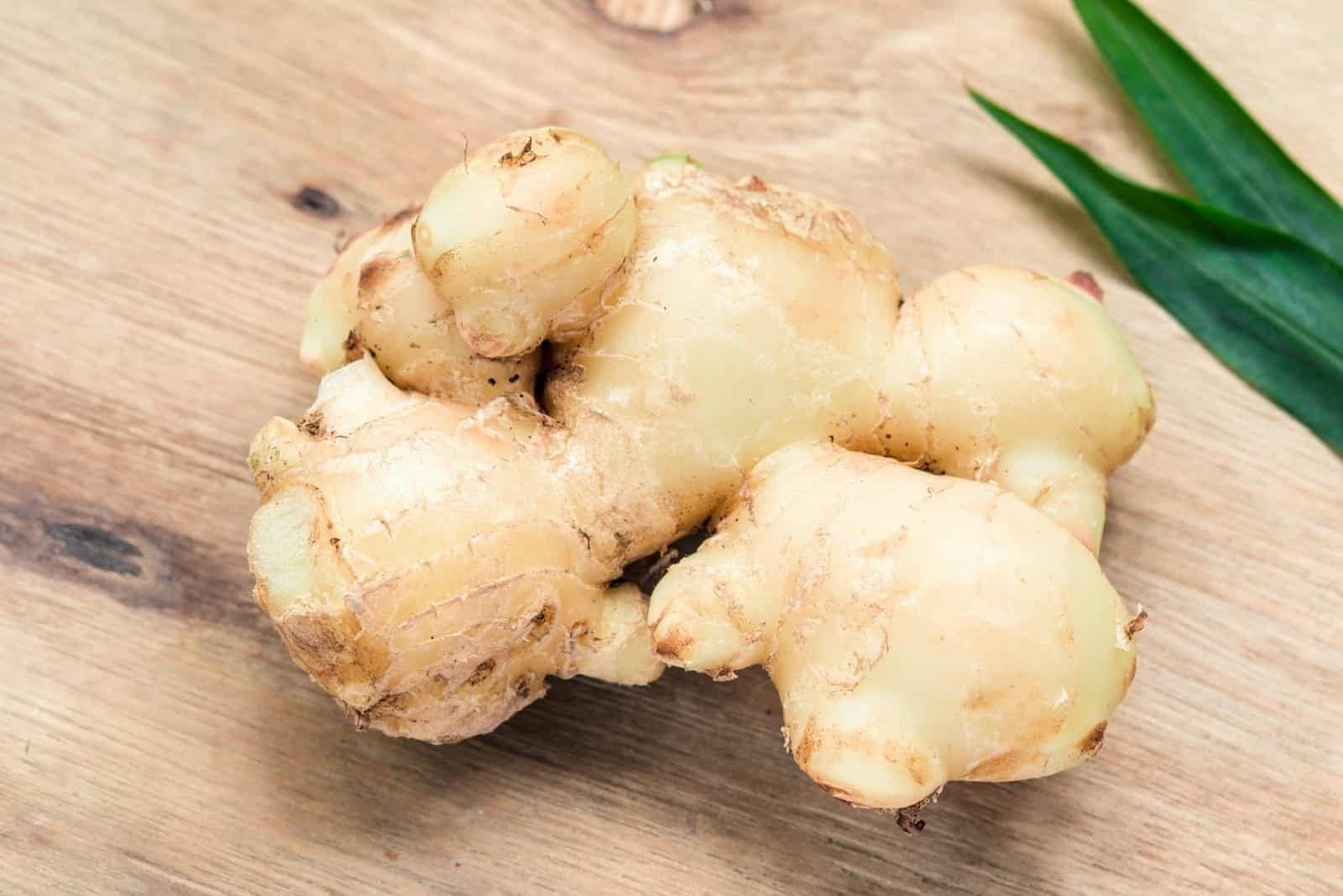
Ginger
This spice has been shown to improve microbiome biodiversity. In a crossover intervention study on 123 healthy subjects (63 men and 60 women) fresh ginger juice increased the species number of intestinal flora within only a week. A decreased relative abundance of the Prevotella-to-Bacteroides ratio and pro-inflammatory Ruminococcus-1 and Ruminococcus-2 while a tendency toward an increased Firmicutes-to-Bacteroidetes ratio, Proteobacteria and anti-inflammatory Faecalibacterium were found. Interestingly significant differences were found between these responses in men and women (70). Evidence of relief of antibiotic-induced diarrhoea as available in other models (71).
Mustard glycosides (glucosinolates)
These sulfur-containing acrids require metabolism by the enzyme myrosinase to yield active isothiocyanates, the health-promoting agents from brassica vegetables like broccoli. However cooking inactivates this enzyme in the plant. Recently several studies have showed that the human gut microbiome can provide the necessary myrosinase, although in variable amounts so that individual responses to these plants are confirmed (72,73).
One important isothiocyanate is sulforaphane, a powerful modulator of inflammation sourced especially in broccoli. It down-regulates Toll-like receptor (TLR) 4 activity reducing bacterial induced activation of inflammatory processes (74)
Garlic
Patients with arterial hypertension who received aged garlic extract increased the composition and diversity of gut bacteria, with an increase in Lactobacillus and Clostridium species and modification in Firmicutes/Bacteroidetes ratio associated with improved health (75).
The traditional garlic intensive approach to gut dysbiosis might be justified! Take a clove of raw garlic, repeating at 30min intervals until at least 8 cloves, through an evening after a day of restricted eating. Treat the following day as if managing a fever: stay warm, drink lots of water. Wash yourself and your sheets and clothes the following day!
Saponins
These detergent principles in plants have long had traditional uses in supporting digestion and intriguingly, given their steroidal structures, also adrenal and reproductive functions. However the whole molecules are again poorly absorbed and their access to body tissues relies on metabolism by gut microbiota (76).
Licorice
Glycyrrhetic acid 3-O-mono-β-D-glucuronide (GAMG), a metabolite with higher bioavailability than the strongly polar parent compound glycyrrhizin, is generated after hydrolysis by β-D- glucuronidase from intestinal bacteria such as Eubacterium sp., Ruminococcus sp., and Clostridium innocuum; they also appear to generate other relevant intestinally absorbed metabolites (such as 3 ⍺-hydroxyglycyrrhetic and 3-oxo-glycyrrhetic acids) with relatively strong pharmacological activities (77).
Glycyrrhiza glabra extract substantially increased propionate-producing species in faecal samples from vegetarians and vegans (Phascolarctobacter, Sutterella; Parasutterella, Cloacibacillus, Desulfovibrio, Roseburia, Bacteroides, Bifidobacterium, Gemmiger) and decreased Eubacterium spp, Enterococcus faecalis, Klebsiella pneumonia) (78).
Ginseng
Accumulating evidence has led ginsenosides, its active saponins, being termed ‘prodrugs’. When orally ingested they pass through the stomach, small intestine and into the large intestine without decomposition by either gastric juice or liver enzymes. Colonic bacteria cleave the oligosaccharide connected to the aglycone stepwise from the terminal sugar to generate the major metabolites, 20S-protopanaxadiol and 20S-protopanaxatriol. These are further activated by intestinal bacterial deglycosylation and fatty acid esterification: diol-type ginsenosides are mainly transformed into compound K and ginsenoside Rh2; triol-type ginsenosides to ginsenoside Rh1 and protopanaxatriol. These metabolites exhibit stronger antitumor, anti-inflammatory, antidiabetic, antiallergic, and neuroprotective effects compared with the parent ginsenosides and are also sustained longer in the body (79,80,81).
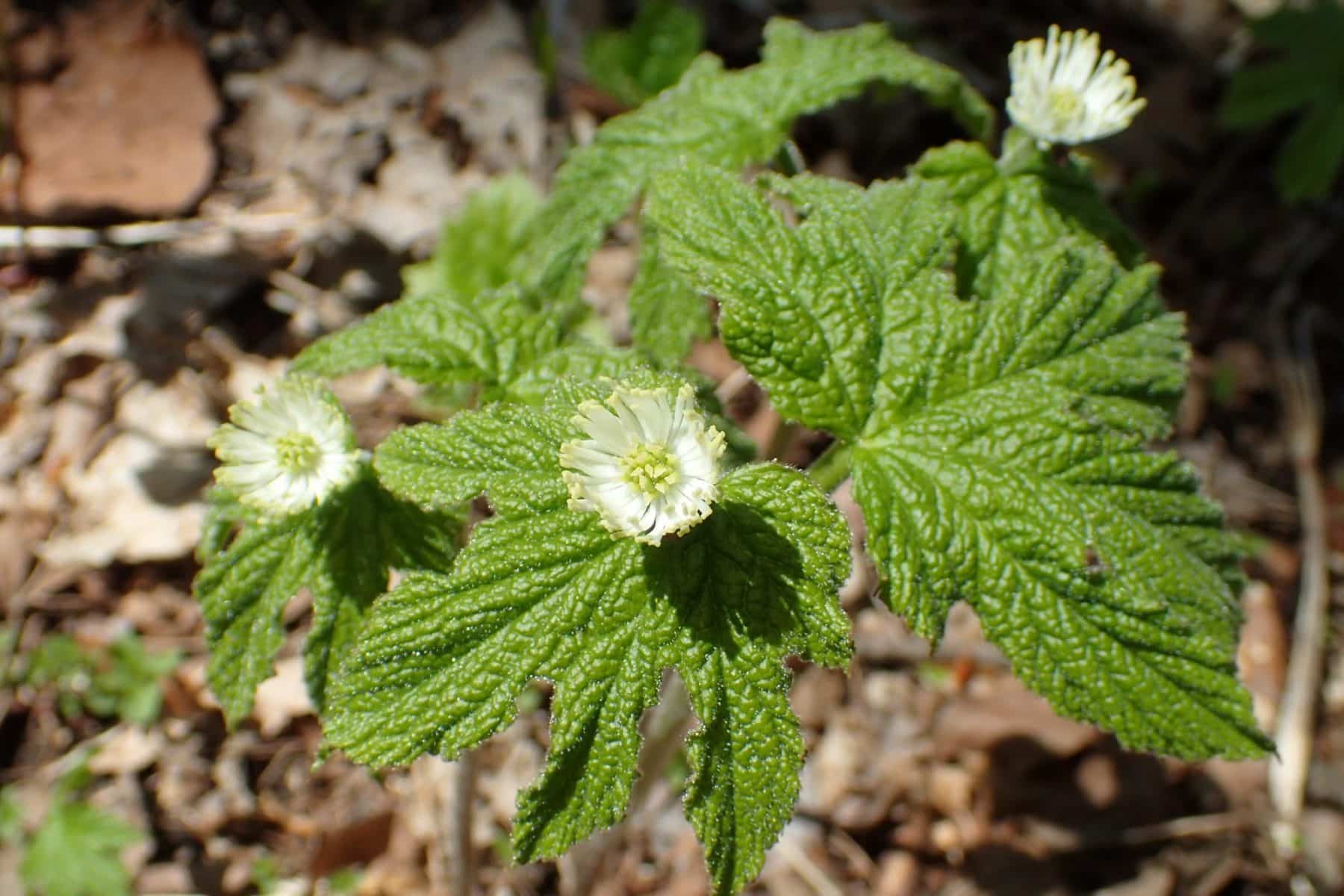
Bitters
Given the key role bitter remedies play in herbal treatments for digestive disorders, it is not surprising that as well as on the taste buds in the mouth, bitter receptors (T2Rs – aka ‘danger receptors’) are found in particular concentrations in the colon. This suggests that they are likely to play a part in host-microbiome interactions, and there is particular interest in their putative role in managing metabolic rate (82).
There is evidence that the effects of the bitter alkaloid berberine, found in barberry, golden seal, Phellodendron, Tinospora and Coptis species, interacts with bacterial species found most commonly in those eating high fat diets (83).
Anthraquinones
The laxative constituents in plants like senna, cascara sagrada have long been known to require microbial metabolism, and hence some hours delay, before they become effective. This has been confirmed (84). An anthraquinone preparation from rhubarb appears to improve gut barrier integrity by promoting mucin-supporting bacteria and other prebiotic effects (85).
Practicalities
What have we learned so far?
The first point to make is that the science and evidence here is only just emerging. An important European review paper confirms that the information gleaned here is still largely speculative and research challenges are daunting (86). However, the pace of discovery is dazzling and the weight of evidence increasingly encourages us to use the plant foods, spices and herbs (87), that we already know have other health benefits, with the likelihood that these are mediated in part, even large part, by their interactions with the gut microbiota.

Dosages
There are immediate logistic challenges in applying plant ingredients to supporting a healthy gut microbiome. The overall weight of this microbial universe is as much as 5 kg and it lives at the back end of a 7-metre tube. To reach this will either require food-level doses, or the activation of reflex activity from triggering receptors higher up the tract. It is simply impractical to expect the average herbal or supplement dose physically to impact the colonies lower in the gut. As an indication, each day our small intestine receives over six litres of liquid from gut secretions (including 4-5 litres of gastric secretions). Three teaspoons of liquid herbs would make less than 1/400 (0.25%) of this total!
It is also important that if direct contact is required, then the constituents confirmed should not be metabolized or absorbed higher up the tract. This is a case when bioavailability is not important.
In effect, the best ways to impact the gut microbiome are
- by using plant constituents that survive digestion to reach the gut in sufficient quantities and then consume these in food-level quantities
- fibre, gums and mucilages
- polyphenols and tannins
- spices
- by triggering responses (e.g. digestive and biliary secretions via upper gut wall receptors) in which case lower doses are achievable. Potential constituents include
- bitters
- acrids
Take-home lessons
We may agree that for a healthy microbiome
- the best diets are those with the widest diversity of plant ingredients, to include a range of phytonutrient-rich foods, spices and herbs, in addition to fermented and other probiotic foods
- the use of antibiotics should be minimised
- strategies should be more akin to the organic gardener’s management of the soil than interventionist manipulations
It is also clear that what we know about the microbiome has already transformed the rules of medicine and that it is possible that much of the activity of our herbal remedies is mediated by their relationship with these organisms within us.
References
- Turnbaugh PJ, Quince C, J. Faith JJ et al., (2010) Organismal, genetic, and transcriptional variation in the deeply sequenced gut microbiomes of identical twins. Proceedings of the National Academy of Sciences of the United States of America. 107, no.16, pp. 7503–7508, 2010
- Guarner F, Malagelada JR. (2003) Gut flora in health and disease. Lancet 361: 512–9 doi: 10.1016/S0140-6736(03)12489-0.
- Rousseaux C, Thuru X, Gelot A, et al. (2007) Lactobacillus acidophilus modulates intestinal pain and induces opioid and cannabinoid receptors. Nat Med. 13(1): 35-7
- Simpson CA, Diaz-Arteche C, Eliby D, et al. (20210 The gut microbiota in anxiety and depression – A systematic review. Clin Psychol Rev. 83: 101943. doi: 10.1016/j.cpr.2020.101943.
- Bouter KE, van Raalte DH, Groen AK, Nieuwdorp M. (2017) Role of the Gut Microbiome in the Pathogenesis of Obesity and Obesity-Related Metabolic Dysfunction. Gastroenterology. 152(7): 1671-1678. doi: 10.1053/j.gastro.2016.12.048.
- Harsch IA, Konturek PC. (20180 The Role of Gut Microbiota in Obesity and Type 2 and Type 1 Diabetes Mellitus: New Insights into “Old” Diseases. Med Sci (Basel). 6(2): 32. doi: 10.3390/medsci6020032
- Forslund K, Hildebrand F, Nielsen T, et al. (2015) Disentangling type 2 diabetes and metformin treatment signatures in the human gut microbiota. Nature. 528(7581): 262-266. doi: 10.1038/nature15766.
- Barrio C, Arias-Sánchez S, Martín-Monzón I. (2022) The gut microbiota-brain axis, psychobiotics and its influence on brain and behaviour: A systematic review. Psychoneuroendocrinology. 137:105640. doi: 10.1016/j.psyneuen.2021.105640
- Aziz Q, Doré J, A. Emmanuel A, et al, (2013) Gut microbiota and gastrointestinal health: current concepts and future directions. Neurogastroenterology and Motility, 25, 1: 4–15
- Hempel S, Newberry SJ, Maher AR, et al. (2012) Probiotics for the prevention and treatment of antibiotic-associated diarrhea: a systematic review and meta-analysis. JAMA. 307(18): 1959-69. doi: 10.1001/jama.2012.3507
- Oak SJ, Jha R. (2019) The effects of probiotics in lactose intolerance: A systematic review. Crit Rev Food Sci Nutr. 59(11): 1675-1683. doi: 10.1080/10408398.2018.1425977.
- Dale HF, Rasmussen SH, Asiller ÖÖ, Lied GA. (2019) Probiotics in Irritable Bowel Syndrome: An Up-to-Date Systematic Review. Nutrients. 11(9): 2048. doi: 10.3390/nu11092048.
- Collinson S, Deans A, Padua-Zamora A, et al. (2020) Probiotics for treating acute infectious diarrhoea. Cochrane Database Syst Rev. 12(12): CD003048. doi: 10.1002/14651858.CD003048.pub4
- Cook MT, Tzortzis G, Charalampopoulos D, Khutoryanskiy VV. (2012) Microencapsulation of probiotics for gastrointestinal delivery. J Control Release. 162(1): 56-67. doi: 10.1016/j.jconrel.2012.06.003.
- Fehlbaum S, Prudence K, Kieboom J, et al. (2018) In Vitro Fermentation of Selected Prebiotics and Their Effects on the Composition and Activity of the Adult Gut Microbiota. Int J Mol Sci. 19(10):3097. doi: 10.3390/ijms19103097
- Whelan K, Judd PA, Preedy VR, et al.(2005) Fructooligosaccharides and fiber partially prevent the alterations in fecal microbiota and short-chain fatty acid concentrations caused by standard enteral formula in healthy humans. J Nutr. 135(8): 1896-902. doi: 10.1093/jn/135.8.1896
- Ojo O, Feng QQ, Ojo OO, Wang XH. (2020) The Role of Dietary Fibre in Modulating Gut Microbiota Dysbiosis in Patients with Type 2 Diabetes: A Systematic Review and Meta-Analysis of Randomised Controlled Trials. Nutrients. 12(11):3239. doi: 10.3390/nu12113239
- McLoughlin RF, Berthon BS, Jensen ME, et al. (2017) Short-chain fatty acids, prebiotics, synbiotics, and systemic inflammation: a systematic review and meta-analysis. Am J Clin Nutr. 106(3): 930-945. doi: 10.3945/ajcn.117.156265.
- Walker AW, Lawley TD (2013) Therapeutic modulation of intestinal dysbiosis. Pharmacological Research; 69: 75–86
- Canfora EE, Meex RCR, Venema K, Blaak EE. (2019) Gut microbial metabolites in obesity, NAFLD and T2DM. Nat Rev Endocrinol. 15(5): 261-273. doi: 10.1038/s41574-019-0156-z
- J.P. Ouwerkerk et al. (2013) Glycobiome: Bacteria and mucus at the epithelial interface. Best Practice & Research Clinical Gastroenterology 27: 25–38
- Kuwahara A, Matsuda K, Kuwahara Y, et al. (2020) Microbiota-gut-brain axis: enteroendocrine cells and the enteric nervous system form an interface between the microbiota and the central nervous system. Biomed Res. 41(5): 199-216. doi: 10.2220/biomedres.41.199.
- Tsuda T. (2015) Possible abilities of dietary factors to prevent and treat diabetes via the stimulation of glucagon-like peptide-1 secretion. Mol Nutr Food Res. 59(7): 1264-73. doi: 10.1002/mnfr.201400871.
- Posovszky C, Wabitsch M. (2015) Regulation of appetite, satiation, and body weight by enteroendocrine cells. Part 1: characteristics of enteroendocrine cells and their capability of weight regulation. Hormone Research in Paediatrics 83.1: 1-10.
- Braun T, Voland P, Kunz L et al. (2007) Enterochromaffin Cells of the Human Gut: Sensors for Spices and Odorants. Gastroenterlogy;132:1890-1901
- Vegezzi G, Anselmi L, Huynh J, et al. (2014) Diet-Induced Regulation of Bitter Taste Receptor Subtypes in the Mouse. Gastrointestinal Tract. PLoS ONE 9(9): e107732
- Pluznick JL, Protzko RJ, Gevorgyan H, et al. (2013) Olfactory receptor responding to gut microbiota-derived signals plays a role in renin secretion and blood pressure regulation. Proc Natl Acad Sci USA. 110(11): 4410-5
- Cherta-Murillo A, Pugh JE, Alaraj-Alshehhi S, et al. (2022) The effects of SCFAs on glycemic control in humans: a systematic review and meta-analysis. Am J Clin Nutr. 116(2): 335-361. doi: 10.1093/ajcn/nqac085.
- Tran SM, Mohajeri MH. (2021) The Role of Gut Bacterial Metabolites in Brain Development, Aging and Disease. Nutrients. 13(3): 732. doi: 10.3390/nu13030732.
- Wahlström A, Sayin SI, Marschall HU, Bäckhed F. (2016) Intestinal Crosstalk between Bile Acids and Microbiota and Its Impact on Host Metabolism. Cell Metab. 24(1):41-50. doi: 10.1016/j.cmet.2016.05.005
- Porez G, Prawitt J, Gross B, Staels B. (2012) Bile acid receptors as targets for the treatment of dyslipidemia and cardiovascular disease. J Lipid Res 53(9): 1723-37.
- Aguilar-Toalá JE, Garcia-Varela R, Garcia HS, et al. (2018) Postbiotics: An evolving term within the functional foods field, Trends in Food Science & Technology, 75: 105-114 doi: /10.1016/j.tifs.2018.03.009
- Salminen, S., Collado, M.C., Endo, A. et al. (2021) The International Scientific Association of Probiotics and Prebiotics (ISAPP) consensus statement on the definition and scope of postbiotics. Nat Rev Gastroenterol Hepatol 18, 649–667 doi:10.1038/s41575-021-00440-6
- https://www.health.harvard.edu/nutrition/what-are-postbiotics
- https://prebioticassociation.org/prebiotic-resources/
- An X, Bao Q, Di S, et al, (2019) The interaction between the gut Microbiota and herbal medicines. Biomedicine & Pharmacotherapy. 118, 109252 doi:/10.1016/j.biopha.2019.109252
- Tomova A, Bukovsky I, Rembert E, et al. (2019) The Effects of Vegetarian and Vegan Diets on Gut Microbiota. Front Nutr. 6:47. doi: 10.3389/fnut.2019.00047
- Zimmer J, Lange B, Frick JS, et al. (2012) A vegan or vegetarian diet substantially alters the human colonic faecal microbiota. Eur J Clin Nutr. 66(1):53-60. doi: 10.1038/ejcn.2011.141
- Lu QY, Summanen PH, Lee RP, et al. (2017) Prebiotic potential and chemical composition of seven culinary spice extracts. J Food Sci. 82:1807-1813. doi:10.1111/1750-3841.13792
- Zhang HY, Tian JX, Lian FM, et al. (2021) Therapeutic mechanisms of traditional Chinese medicine to improve metabolic diseases via the gut microbiota. Biomed Pharmacother. 133: 110857. doi: 10.1016/j.biopha.2020.110857.
- Li Y, Ji X, Wu H, et al. (2021) Mechanisms of traditional Chinese medicine in modulating gut microbiota metabolites-mediated lipid metabolism. Journal of Ethnopharmacology 278, 114207. doi:/10.1016/j.jep.2021.114207
- Zhong P, Zhou J, Fan YT, et al. (2022) Co-existing polysaccharides affect the systemic exposure of major bioactive ingredients in Chang-Kang-Fang, a multi-herb prescription for treatment of irritable bowel syndrome. J Ethnopharmacol. 298: 115601. doi: 10.1016/j.jep.2022.115601
- Calame W, Weseler AR, Viebke C, et al. (2008) Gum arabic establishes prebiotic functionality in healthy human volunteers in a dose-dependent manner. Br J Nutr. 100(6):1269-75. doi: 10.1017/S0007114508981447.
- Kamal E, Kaddam LA, Dahawi M, et al. (2018) Gum Arabic Fibers Decreased Inflammatory Markers and Disease Severity Score among Rheumatoid Arthritis Patients, Phase II Trial. Int J Rheumatol. 2018:4197537. doi: 10.1155/2018/4197537
- Kawabata K, Yoshioka Y, Terao J. (2019) Role of Intestinal Microbiota in the Bioavailability and Physiological Functions of Dietary Polyphenols. Molecules. 24(2): 370. doi: 10.3390/molecules24020370.
- Mithul Aravind S, Wichienchot S, Tsao R, et al. (2021) Role of dietary polyphenols on gut microbiota, their metabolites and health benefits. Food Res Int. 142:110189. doi: 10.1016/j.foodres.2021.110189
- Koudoufio M, Desjardins Y, Feldman F, et al. (2020) Insight into Polyphenol and Gut Microbiota Crosstalk: Are Their Metabolites the Key to Understand Protective Effects against Metabolic Disorders? Antioxidants (Basel). 9(10):982. doi: 10.3390/antiox9100982.
- Puupponen-Pimiä R, Nohynek L, Hartmann-Schmidlin S, et al. (2005) Berry phenolics selectively inhibit the growth of intestinal pathogens. J Appl Microbiol. 98(4): 991-1000. doi: 10.1111/j.1365-2672.2005.02547
- Barański M, Srednicka-Tober D, Volakakis N, et al. (2014) Higher antioxidant and lower cadmium concentrations and lower incidence of pesticide residues in organically grown crops: a systematic literature review and meta-analyses. Br J Nutr. 112(5):794-811. doi: 10.1017/S0007114514001366.
- Daniel O, Meier MS, Schlatter J, Frischknecht P. (1999) Selected phenolic compounds in cultivated plants: ecologic functions, health implications, and modulation by pesticides. Environ Health Perspect. 107 (Suppl 1):109-14. doi: 10.1289/ehp.99107s1109.
- Sorrenti V, Ali S, Mancin L, et al. (2020) Cocoa Polyphenols and Gut Microbiota Interplay: Bioavailability, Prebiotic Effect, and Impact on Human Health. Nutrients. 27;12(7):1908. doi: 10.3390/nu12071908
- Pérez-Burillo S, Navajas-Porras B, López-Maldonado A, et al. (2021) Green Tea and Its Relation to Human Gut Microbiome. Molecules. 26(13): 3907. doi: 10.3390/molecules26133907.
- Chen H., Sang S. (2014) Biotransformation of tea polyphenols by gut microbiota. J. Funct. Foods. 7:26–42. doi: 10.1016/j.jff.2014.01.013
- Zam W. (2018) Gut Microbiota as a Prospective Therapeutic Target for Curcumin: A Review of Mutual Influence. J Nutr Metab. 2018:1367984. doi: 10.1155/2018/1367984
- McFadden RM, Larmonier CB, Shehab KW, et al. (2015) The role of curcumin in modulating colonic microbiota during colitis and colon cancer prevention. Inflamm Bowel Dis. 21: 2483–94
- Shimouchi A, Nose K, Takaoka M, et al. (2009) Effect of dietary turmeric on breath hydrogen. Dig Dis Sci. 54 8: 1725-9
- Alli-Oluwafuyi AM, Luis PB, Nakashima F, et al. (2019) Curcumin induces secretion of glucagon-like peptide-1 through an oxidation-dependent mechanism. Biochimie. 165: 250-257. doi: 10.1016/j.biochi.2019.08.013.
- Panaro MA, Corrado A, Benameur T, et al. (2020) The Emerging Role of Curcumin in the Modulation of TLR-4 Signaling Pathway: Focus on Neuroprotective and Anti-Rheumatic Properties. Int J Mol Sci. 26; 21(7):2299. doi: 10.3390/ijms21072299
- Peterson CT, Vaughn AR, Sharma V, et al. (2018) Effects of Turmeric and Curcumin Dietary Supplementation on Human Gut Microbiota: A Double-Blind, Randomized, Placebo-Controlled Pilot Study. Journal of Evidence-Based Integrative Medicine 23: 1-8 doi: 10.1177/2515690X18790725
- Shen L, Ji HF. (2018) Bidirectional interactions between dietary curcumin and gut microbiota. Crit Rev Food Sci Nutr. 59(18):2896-2902. doi: 10.1080/10408398.2018.1478388
- Marciani L, Cox EF, Hoad CL et al (2013). Effects of various food ingredients on gall bladder emptying. Eur J Clin Nutr. 11, 1182-7
- Dey N, Wagner VE, Blanton LV (2015) Regulators of gut motility revealed by a gnotobiotic model of diet-microbiome interactions related to traveling. Cell 163, 1, 95-107
- Wang Y, Wang L, Zhu X et al (2016) Choleretic Activity of Turmeric and its Active Ingredients. Journal of Food Science 81, 7, 1800-06.
- Hu Q, Zhang W, Wu Z, et al. (2021) Baicalin and the liver-gut system: Pharmacological bases explaining its therapeutic effects. Pharmacol Res. 165:105444. doi: 10.1016/j.phrs.2021.105444
- Han K, Bose S, Wang JH, et al. (2017) In vivo therapeutic effect of combination treatment with metformin and Scutellaria baicalensis on maintaining bile acid homeostasis. PLoS One. 12(9): e0182467. doi: 10.1371/journal.pone.0182467.
- Kawabata K, Yoshioka Y, Terao J. (2019) Role of Intestinal Microbiota in the Bioavailability and Physiological Functions of Dietary Polyphenols. Molecules. 24(2): 370. doi: 10.3390/molecules24020370.
- Piwowarski JP, Granica S, Zwierzyńska M, et al (2014) Role of human gut microbiota metabolism in the anti-inflammatory effect of traditionally used ellagitannin-rich plant materials. Journal of Ethnopharmacology. 155 (1): 801-809. doi./10.1016/j.jep.2014.06.032
- Rosca AE, Iesanu MI, Zahiu CDM, et al. (2020) Capsaicin and Gut Microbiota in Health and Disease. Molecules. 25(23):5681. doi: 10.3390/molecules25235681
- Kang C, Zhang Y, Zhu X, et al. (2016) Healthy Subjects Differentially Respond to Dietary Capsaicin Correlating with Specific Gut Enterotypes. J Clin Endocrinol Metab. 101(12): 4681-4689. doi: 10.1210/jc.2016-2786.
- Wang X, Zhang D, Jiang H, et al. (2021) Gut Microbiota Variation With Short-Term Intake of Ginger Juice on Human Health. Front Microbiol. 11:576061. doi: 10.3389/fmicb.2020.576061.
- Ma ZJ , Wang HJ , Ma XJ , et al. (2020) Modulation of gut microbiota and intestinal barrier function during alleviation of antibiotic-associated diarrhea with Rhizoma Zingiber officinale (Ginger) extract. Food Funct. 11(12): 10839-10851. doi: 10.1039/d0fo01536a
- Sikorska-Zimny K, Beneduce L. (2021) The Metabolism of Glucosinolates by Gut Microbiota. Nutrients. 13(8): 2750. doi: 10.3390/nu13082750.
- Tian S, Liu X, Lei P, et al. (2018) Microbiota: a mediator to transform glucosinolate precursors in cruciferous vegetables to the active isothiocyanates. J Sci Food Agric. 98(4): 1255-1260. doi: 10.1002/jsfa.8654.
- Youn HS, Kim YS, Park ZY, et al. (2010) Sulforaphane suppresses oligomerization of TLR4 in a thiol-dependent manner. J Immunol. 184(1):411-9. doi: 10.4049/jimmunol.0803988.
- Ried K, Travica N, Sali A. (2018) The Effect of Kyolic Aged Garlic Extract on Gut Microbiota, Inflammation, and Cardiovascular Markers in Hypertensives: The GarGIC Trial. Front Nutr. 5:122. doi: 10.3389/fnut.2018.00122
- Luo Z, Xu W, Zhang Y, Di L, Shan J. (2020) A review of saponin intervention in metabolic syndrome suggests further study on intestinal microbiota. Pharmacol Res. 160: 105088. doi: 10.1016/j.phrs.2020.105088.
- Zhang F, He F, Li L, et al. (2020). Bioavailability based on the gut microbiota: a new perspective. Microbiol Mol Biol Rev 84:e00072-19. doi.org/10 .1128/MMBR.00072-19
- Peterson CT, Sharma V, Uchitel S, et al (2018) Prebiotic potential of herbal medicines used in digestive health and disease J. Altern. Complement. Med., 24 (2018), pp. 656-665
- Kim DH. (2018) Gut microbiota-mediated pharmacokinetics of ginseng saponins. J Ginseng Res. 2018 Jul;42(3):255-263. doi: 10.1016/j.jgr.2017.04.011
- Hasegawa H. (2004) Proof of the mysterious efficacy of ginseng: basic and clinical trials: metabolic activation of ginsenoside: deglycosylation by intestinal bacteria and esterification with fatty acid. J Pharmacol Sci. 95(2): 153-7
- Yang L, Zou H, Gao Y, et al. (2020) Insights into gastrointestinal microbiota-generated ginsenoside metabolites and their bioactivities. Drug Metab Rev. 52(1): 125-138. doi: 10.1080/03602532.2020.1714645.
- Turner A, Veysey M, Keely S, et al. (2018) Interactions between Bitter Taste, Diet and Dysbiosis: Consequences for Appetite and Obesity. Nutrients. 10(10):1336. doi: 10.3390/nu10101336.
- Wang Y, Tong Q, Shou JW, et al. (2017) Gut Microbiota-Mediated Personalized Treatment of Hyperlipidemia Using Berberine. Theranostics. 7(9): 2443-2451. doi: 10.7150/thno.18290.
- Huang Z, Xu Y, Wang Q, Gao X. (2019) Metabolism and mutual biotransformations of anthraquinones and anthrones in rhubarb by human intestinal flora using UPLC-Q-TOF/MS. J Chromatogr B Analyt Technol Biomed Life Sci. 1104: 59-66. doi: 10.1016/j.jchromb.2018.10.008
- Cui HX, Zhang LS, Luo Y, et al. (2019) A Purified Anthraquinone-Glycoside Preparation From Rhubarb Ameliorates Type 2 Diabetes Mellitus by Modulating the Gut Microbiota and Reducing Inflammation. Front Microbiol. 10: 1423. doi: 10.3389/fmicb.2019.01423.
- Thumann TA, Pferschy-Wenzig EM, Moissl-Eichinger C, Bauer R. (2019) The role of gut microbiota for the activity of medicinal plants traditionally used in the European Union for gastrointestinal disorders. J Ethnopharmacol. 245:112153. doi: 10.1016/j.jep.2019.112153
- Chen F, Wen Q, Jiang J, et al. (2016) Could the gut microbiota reconcile the oral bioavailability conundrum of traditional herbs? J Ethnopharmacol. 179: 2